In the renewable energy solutions in the building sector for the energy transition, we too often forget the significant potential offered by geothermal heat pumps in the tertiary, collective, and even individual sectors...[1] This is what is known as geo-energy, a very specific category of geothermal energy known as “surface geothermal energy”. It offers a very efficient solution for the thermal renovation of buildings, in particular to meet the needs of air conditioning which will increase due to global warming. Indeed, the thermal inertia of the subsoil, both cool in summer and warm in winter, can be exploited by this means, and assisted by heat pumps. This local geo-energy, decarbonated, renewable and neutral for the landscape is still far from having realized its potential in areas where its massification would be more than relevant.
Among the energy solutions aiming at both mitigation and adaptation to climate change, geothermal energy assisted by geothermal heat pumps has an important role to play, especially in the field of space heating and cooling for new buildings, but also in thermal renovation strategies as climate change is expected to become a reality, and in particular the multiplication of heat waves (Read: Geothermal energy: an important hidden resource). According to the French High Council for the Climate[2], it is essential not to limit ourselves to thermal renovation in the strict sense of the word, but to work on energy and even climate renovations (Read: Renewable Heat and Thermal Rehabilitation of Housing, Two Complementary Paths for the Low Carbon Transition). The difference is significant: in an energy renovation, we also integrate the decarbonization of the energy source itself; in a climate renovation, we take into account climate change, and therefore the growing need for cooling.
After having clarified the functioning of this technology, we explain its environmental benefits which should justify its more massive integration in the French and even more widely European energy mix. Indeed, with the exception of some precursor countries such as Switzerland or Sweden, Europe is lagging behind Asia or America[3].
1. Geo-energy, a particular form of geothermal energy
Etymologically, geothermal energy comes from the ancient Greek γῆ (guê) earth and θερμός (thermos) which means hot: geothermal energy thus naturally refers to what is called deep geothermal energy, and which consists in extracting heat from the decay of radioactive rocks in the subsoil. Depending on the depth, this heat can be used either directly or to produce electricity.
- In the Paris region, heating networks for buildings and swimming pools have been using the water from the Dogger[4] since the 1970s: this formation, located at a depth of about 1.5 km, makes it possible to exploit the thermal potential of water at 70°C. This is called low enthalpy or low temperature geothermal energy.
- The use of deep geothermal energy to produce electricity was unfortunately illustrated in the recent news with the earthquake felt near Strasbourg at the end of 2020. In this case, even if Alsace benefits from a high geothermal gradient, it is still necessary to drill 5km away to have a temperature of 150°C.
The technology we are dealing with in this article is often referred to as “geothermal energy”, which is a bit of a departure from its etymology because its purpose is not to extract heat from the earth. Indeed, at the depths at which the drillings are carried out, typically a few tens of meters, the ground temperature is close to the average seasonal temperature. This constant temperature, between 12°C and 15°C in France, is cooler than the outside air in summer and warmer in winter. And it is this tempered value that makes it so interesting for the thermal comfort of a building, which will increasingly need to be cooled in summer, and not only heated in winter.
Thus, we prefer to refer to it by the word geo-energy which is of more neutral etymology, ἐνέργεια / enérgeia meaning “force in action” in ancient Greek. In addition to its different principle, geo-energy requires much more compact drills for its implementation than those of oil rigs used in deep geothermal energy. It also does not use hydraulic fracturing techniques such as those used in Alsace are never used.
2. Geo-energy techniques
Between the exploitation of the subsoil temperature and the heating or cooling of a room, several techniques are implemented.
2.1. The part of the techniques in the underground
Two techniques, illustrated in figure 1 below, are classically used to exploit the constant temperature of the underground.
The first one is only possible if the basement of the building is above a water table, which is usually the case in city centers. It consists in drilling two wells of typically 25 to 50 cm in diameter, one used to extract water from the water table, and the other to return it after having taken its energy via an exchanger (Read: Heat exchangers). Legislation requires that all the water extracted be returned, so as not to dry up the water table. These boreholes can easily be made in dense areas, sometimes in parking lots. With this principle, it is the natural flow of water in the rock that allows its thermalization, hence the term “open system”. An estimate of the power and energy available thanks to these aquifers for the Paris region was carried out by the Bureau de Recherches Géologiques et Minières (BRGM) for ADEME in 2012[6] :in relation to the needs of buildings, it is estimated at 20,000GWh/year (about 1.7 Mtep/year) for the Ile de France, in the “2020 scenario”.
The second technique, “closed systems”, consists in artificially creating a heat exchanger with the subsoil. This consists in making several drillings, called “probes”, of 15 cm in diameter. Each of these boreholes contains a U-shaped tube, lined with cement. Glycolated water is circulated through these tubes, the glycol being used to prevent the water from freezing in the event of sub-zero temperatures. On this diagram, we illustrate the configuration where the boreholes are vertical, which allows to maximize the exchanges with the subsoil, thus the extracted energy. They must be spaced 10m apart so that they do not interact with each other, which is why this technology is typically used away from city centers, in areas where there is little groundwater.
Note that other geometries are possible, some of which are under development:
- Diverted drilling when the entire subsoil is not directly accessible, inspired by oil drilling that starts from the same point, and allowing to exploit closed systems even in dense urban areas;
- Underground exchangers in the form of “baskets” or “walls” for smaller buildings, which do not require a drill for their installation;
- Horizontal exchangers in which air is usually circulated, which do not require a drill either: they require a plot of land twice the size of the building to be heated/cooled – which explains their greater development in Canada than in European cities; they are called “Canadian wells”…
2.2. Techniques on the building side (heat pump and emitters)
Whatever the technology used in the basement, the transfer of calories from the “primary loop”, which extracts the energy from the basement, is done via an exchanger: the ground water never circulates throughout the building. After this exchanger, a heat pump is used, usually reversible, to bring the water from 12-15°C to the temperature regime suitable for the building.
This temperature regime is important because the lower the temperature difference between the cold and hot sources, the higher the efficiency of the heat pumps. In fact, to fix ideas, we will call some basic notions of thermodynamics: a heat pump is a device allowing, with the help of work W, to transfer heat from a cold source, which exchanges a quantity of heat Qf at the temperature Tf, to a hot source, which exchanges a quantity of heat Qc at the temperature Tc. (Read: Sadi Carnot facing the end of the thermo-industrial civilization, Thermodynamics: energy and entropy, Thermodynamics: the laws). We then deduce directly from the writing of the first two principles that the yield (Carnot’s ideal), quotient of Qc by W, is inversely proportional to the difference in temperatures Tc-Tf:
This specificity of heat pumps has two consequences that must be underlined:
- First of all, for the same building, a geothermal heat pump is more efficient than an aerothermal heat pump, because the underground temperature is higher than the air temperature in winter and cooler in summer.
- In addition, a heat pump system is more efficient the lower the temperature used to heat the building.
So-called low-temperature “emitters”, such as radiant floors or ceilings, are therefore favored over high-temperature systems, such as old, conventional cast-iron radiators, adapted to boilers running on hydrocarbons. We estimate that the efficiency (or coefficients of performance) are of the order of 400%: with 1kWh of electricity, 4kWh of heat or cold are produced. Higher values are found in the literature[7] (from 800 to 1200%) but these are obtained under particularly favorable conditions, with low temperature emitters, which are not usually found in existing buildings.
In summer, if radiant floors or ceilings are used to cool the building, we can even consider bypassing the heat pump in order to use the cold directly by recovering it through an exchanger (see: Cooling: uses and production). This is known as “free cooling” or “geocooling”, and in this case the efficiency can be as high as 5000% [8] because the electricity consumption is only used to circulate the water.
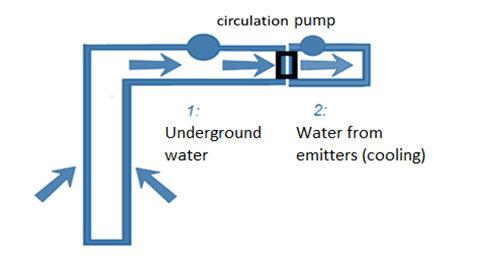
Figure 3: Schematic representation of a free cooling/geo-cooling geothermal system [Source: Geotrainet Manual]
Heat pumps and “emitters” are mature technologies, but more research is needed, especially on refrigerants. Some work is identifying increasingly efficient fluids, to maximize the yields obtained[9] and to use other components than those emitting greenhouse gases such as traditional fluids (notably chlorine hexafluoride HFC and HCFC) which have a very high greenhouse effect (140 to 11,000 times more powerful than CO2). The use of CO2 or even water as refrigerants is being considered.
2.3. Evaluate the performance of the entire system
During a given season, a geo-energy operation tends to change the temperature of the subsoil: in winter it is cooled while in summer it is warmed. If the building’s needs are balanced between hot and cold, their effects cancel each other out over a year. The thermal inertia of the basement allows for inter-seasonal storage, which further increases the available power compared to a scenario in which the ground would not be recharged from one season to the next.
Thus, it is important to couple the thermal models of the basement and the building in order to determine the available resources. This result, which is somewhat counter-intuitive, reminds us of the need for a systemic approach to finely analyze the subsoil. And symmetrically, with a building perspective, it shows that we cannot do without an analysis including the subsoil. It also implies the availability of tools to do so.
Let’s also note the complementarity with other renewable energy sources, depending on the needs of the building. For example, for a residential building that has, to date, exclusively heating needs, and in order to avoid that the basement loses efficiency over time, one can combine the geo-energy installation with a solar thermal installation. The latter can then provide the domestic hot water needs and serve to “recharge” the basement in summer: by dimensioning it for winter, the excess calories produced in summer can be stored in the basement (Read: Cogeneration and seasonal heat storage for residential and commercial applications).
It is also economically interesting to consider a partial coverage of the needs by geo-energy. Indeed, the high power calls, both in heating and cooling, are made over a short period: to maximize the return on investment, it is then generally more interesting to size the installation for a coverage of 50% of the power needs, because it allows to cover more than 80% of the energy needs. The rest of the power is then provided by a complementary installation, in particular an electric heating. This allows an economic optimization which can be an essential lever for some customers.
2.4. Environmental benefits
In addition to energy savings through local production of renewable energy, a geo-energy installation can have other environmental benefits.
First of all, it can reduce greenhouse gas emissions. To make a global carbon balance, we start by estimating the impact of the wells themselves, including their end-of-life (re-capping). For two 65-meter deep wells, this is about 30 teqCO2, with almost half of this for the materials themselves (cement, stainless steel). In operation, it is then necessary to make assumptions on the consumption of the building and its heating/cooling mode. For example, a 10,000m2 building with an initial consumption of 3.15GWh/year is reduced to 0.8 GWh/year with geo-energy covering all the needs. If the building was initially heated with natural gas and cooled with a conventional air conditioner, the emissions would drop from 500 teqCO2/year to 65 teqCO2/year, a reduction of 87%.
This saving pays for the carbon “cost” of building the wells in a single month. This gain comes from the reduction in consumption (by 75% in this case), and from the fact that fossil gas, which emits 0.25 kgeqCO2/kWh of heat, is not used in favor of electricity, which emits 0.08 kgeqCO2/kWh [10] of electricity in France. It should be noted that this carbon footprint is a function of both the initial energy source installed and the carbon footprint of the electricity. It therefore varies from one building to another, and from one country to another, depending on the electricity mix in place.
The impact of geo-energy on biodiversity is mainly related to drilling. But even with sufficiently deep wells (between 10 and 200 m deep), and remaining within the regulatory framework, we do not expect to significantly disturb ecosystems. Moreover, the fact of burying the means of production allows, in dense areas, not to intensify the phenomena of heat islands contrary to aerothermal systems.
2.5. The economic and social profitability of the projects
The study of geo-energy projects in various types of buildings (residential buildings, offices, shopping centers, schools…) shows satisfactory payback times, as shown in Table 1 below [11].
Table 1. Different cases of geo-energy projects in semi-collective and tertiary buildings
Rouget de L’Isle Residence [12] | Ikea Tourville[13] | PlacoSefalog[14] | |
Type of use | 151 dwellings | Tertiary | Industrial |
Surface | 10 000m2 | 30 000m2 | 7800m2 |
Investment | 300 k€ | 688 k€ | 785k€ |
Return time | 6 years | 8 years | 5 years |
Environmental benefits | 230teqCO2/yearsaved | 630teqCO2/year saved | 103teqCO2/year saved |
It should be noted that short payback times can be obtained for large buildings, even if the geo-energy covers only a part of the energy needs, because it is fully used all year round.
It should also be noted that the elements of financial profitability are not the only parameter of the global profitability of the investment. In fact, in a broader framework that takes into account the real estate value of a building, other arguments can be taken into consideration: the consideration of environmental, social and governance (ESG) or carbon criteria by investors, the transformation of technical roof surfaces into tree terraces, or the silent and invisible nature of the technology.
3. Policies and incentives
Heat represents 42.3% of final energy consumption in France (in 2017); the residential-tertiary sector contributes 65% of consumption and industry 30%. Heat pumps satisfy less than 2% of residential needs, next to the 13% of direct electric heating. The law on “energy transition for green growth” has set a target of 38% of renewable energy in final heat consumption by 2030. What measures should be taken to increase the contribution of heat pumps, and in particular to get geo-energy, which is considered as RE, off the ground in this effort?
3.1. The example of Sweden
The example of the successful development of geothermal heat pumps (GHPs) in Sweden could be used to guide the definition of a favorable policy. The development of heat pumps is not new in Sweden since the first measures date back to the end of the 1970s. It has not experienced the obstacles encountered in France due to the poor performance of installations caused by the lack of skills of installers and manufacturing defects. In Sweden, a solid network of competent installers has been able to develop, particularly in the field of geothermal heat pumps, which account for a third of the total installed capacity of heat pumps, which has maintained a dynamic of success in a very favorable selection environment (policies and measures, technological knowledge and know-how).
In addition to a fairly high carbon tax since the 1990s, Sweden has put in place a set of economic incentives to switch to low-carbon technologies in space heating. This starts with the extensive use of district heating, which is mainly fueled by biofuels from forestry (70%) and municipal waste. Where the development of a district heating network is not possible, high-efficiency heat pumps are widely used in tertiary buildings (16% of the need), multi-family housing (8% of the need) and single-family houses (48% of the need) (Gehlin, Andersson, 2016; IEA, 2019,p.69) [15].One third of the heat pumps work with geothermal heat (“bergvärme” in Swedish) through boreholes a few dozen meters deep.
The effort to deploy heat pumps has been an integral part of the energy renovation of the existing Swedish building stock. This effort has required support through building codes for new buildings, well-defined regulations (connection requirements, etc.) and a number of well-targeted subsidies (e.g., extensive renovation including the installation of heat pumps), not to mention direct public investment in district heating.
3.2. Removing obstacles in France
In order to develop this sector in France, it would first be necessary to link the geo-energy subsidy system to support for thermal renovation of housing, as is the case in Sweden, which is not really the case in France for heat pumps in general. This being said, geothermal energy projects are eligible for aid from the “heat fund” set up to, in the case of surface geothermal energy[16], encourage owners to equip themselves with a geothermal heat pump, by reimbursing the additional cost of drilling compared to a traditional installation.
In addition to the assistance provided by the heat fund, several energy companies offer to finance these installations, earning their income from the sale of heat and cold. In addition, in the case of tertiary sector projects, the reduction in building consumption due to the installation of a geothermal heat pump makes it possible to be eligible for aid under the decree on obligations to reduce final energy consumption in tertiary sector buildings[17].
In order to achieve the government’s objectives for the share of renewable energy in 2030 by relying on geothermal energy, we must also try to remove some obstacles.
- In 2015, the mining code has already been revised to facilitate administrative procedures. In areas that do not present any particular geological risk, and for powers of less than 500kW and depths limited to 200m, it is possible to drill installations with a simple declaration of work. On the other hand, for higher powers or in areas presenting risks, such as certain types of clay formations that swell in the presence of water, it is necessary to request a prefectoral authorization.
- A second obstacle is the lack of knowledge of the potential of geo-energy. To overcome this, the French Agency for the Environment and Energy Management (ADEME), the Renewable Energy Union (SER) [18] and the French Association of Geothermal Professionals (AFPG)[19] are multiplying awareness campaigns.
Conclusion
Why do intermittent renewables have the upper hand over geo-energy? Probably because geo-energy is not seen, even though it represents a significant decarbonization potential, which is too ignored and economically easy to access. There is still a long way to go before a large part of the needs of the residential and tertiary sectors are met, as Sweden has done since 1990, with the large-scale development of heat pumps for heating buildings, one third of which is geo-energy [20]. In France, the sector needs to be structured in terms of legislation, financial aid, communication and technical support for owners
[1] A shorter version of this article was published in French in the Revue de l’Énergie, n°655, March-April 2021 under the title: “La géo-énergie, l’oubliée du mix énergétique français?” It is reproduced here in part with the permission of the Revue de l’Énergie, which we thank.
[2]Renovating better: lessons from Europe – High Council for Climate (hautconseilclimat.fr)
[3]Direct Utilization of Geothermal Energy 2020 Worldwide Review (geothermal-energy.org)
[4]Dogger geothermal energy in Ile-de-France – SIGES Seine-Normandie – ©2021 (brgm.fr)
[5]Geotrainet Training Manual for designers of geothermal systems, Project: IEE/07/581/SI2.499061
[6]BRGM 2012 Étude préalable à l’élaboration du schéma de développement de la géothermie en Île-de-Francehttps://www.enrchoix.idf.ademe.fr/ressources/geothermie/etude-geothermies-idf-srcae.pdf BRGM/ RP-60615-EN
[7]https://www.syndicat-energies-renouvelables.fr/wp-content/uploads/basedoc/guide-methodologique-geothermie-de-surface.pdf
[8]http://www.afpg.asso.fr/wp-content/uploads/2015/04/Residence_tertiaireLePapillon.pdf
[9]Advances in Mechanical Engineering 2019, Vol. 11(1) 1-14
[10]Order of 15 September 2006 on energy performance diagnosis for existing buildings offered for sale in metropolitan France – Légifrance (legifrance.gouv.fr)
[11]https://www.geothermies.fr/viewer/?al=autolayer_operation_surface: By clicking on each of the points represented on the map, one has access to a complete description sheet indicating the investments, their payback time on the decrease of charges, the amount of subsidies obtained, the environmental benefits of the installations.
[12]https://www.geothermies.fr/sites/default/files/inline-files/pantin.pdf
[13]https://www.geothermies.fr/sites/default/files/inline-files/IKEA%20TOURVILLE.pdf
[14]https://www.geothermies.fr/sites/default/files/inline-files/dadonville%20placo.pdf
[15]IEA, 2019, Energy Policies of IEA Countries, The Sweden 2019 Review. Paris: OECD
- Gehlin, O. Andersson, 2016. Geothermal Energy Use, Country, Update for Sweden, https://media.geoenergicentrum.se/2017/02/Gehlin_Andersson_2016_-EGC-2016-CU-Sweden.pdf
[16]https://www.ademe.fr/expertises/energies-renouvelables-enr-production-reseaux-stockage/passer-a-laction/produire-chaleur/fonds-chaleur-bref
f
[17]Decree No. 2019-771 of July 23, 2019 on obligations for actions to reduce final energy consumption in buildings for tertiary use – Légifrance (legifrance.gouv.fr)
[18]Home – Syndicat des énergies renouvelables (syndicat-energies-renouvelables.fr)
[20]Direct Utilization of Geothermal Energy 2020 Worldwide Review (geothermal-energy.org)