Biomass is human primary source of energy, massively available in the world, apparently without limits since based on solar energy. In reality, the use of biomass to satisfy human needs stopped being sustainable decades ago since its extraction did represent a significant part of its regeneration. Under the effect of demographic and economic growth, the massive increase in this extraction is therefore problematic.
For thousands of years, the use of biomass has been sufficient to meet people’s vital needs, providing food, heat, fibers (e.g. clothes) and even shelter. Industrialisation was initially based on wood energy, mostly charcoal: however, the damage to forests was so important, both in China and in Western Europe, that its use was restricted. The massive use of coal, and later oil and natural gas, broke the bottleneck and triggered a dramatic acceleration in the development and material enrichment of some societies.
Since the beginning of the 21st century, humanity has engaged on a global scale to withdraw from using fossil fuels. This energy transition raises countless problems linked to the exploitation of energy sources that are still available, in particular biomass, which use is increasing (Read: Biomass and energy: from primary resources to final energy).
Why do we use biomass? Firstly, to satisfy our vital needs. Although considered negligeable in terms of energy compared to the consumption of fossil fuels, they are not. The most vital of these needs is to eat about 2,500 kilocalories a day: adding up the needs of the 7.8 billion inhabitants of the planet leads to 30×1018 Joules (30 ExaJoules – EJ) a year, or 0.7 billion tonnes of oil equivalent (Gtoe [1]), which represents about 5% of all primary energy produced. To this, many other needs must be added, notably wood energy and biofuels.
The use of biomass has been criticised for its lack of ecological sustainability. The exploitation of a resource is only sustainable if its regeneration is preserved. The important parameters at stake here are therefore the annual production of biomass on the one hand, and the proportion of this production that the world uses on the other hand.
1. From solar flux to net primary productivity (NPP): the fundamental limit of available biomass
The solar flux reaching the top of the atmosphere averages 340 W/m2 for the planet Earth, and 180 W/m2 at its surface, the rest being absorbed or reflected by the atmosphere. This available solar energy is huge, of the order of 3×1024 Joules per year (or 1017 Watts) over the entire surface of the Earth. Most of this flux is absorbed by the Earth’s surface, oceans and continents, and warms it.
A tiny part of this incident flux is captured by photosynthetic organisms to produce organic matter, known as biomass (Read: Photosynthèse et biomasse) at a rate of about 220 billion tonnes of carbon per year (GtC/year). This production is expressed here as a carbon flow because photosynthesis draws CO2 from the air and convert it into organic molecules. This production can also be considered as a flow of energy, available in the produced biomass: in fact, all living organisms draw the energy necessary for their functioning (metabolism) from this biomass via the process of respiration, or sometimes fermentation (Read: Conversion de l’énergie chimique des plantes en énergie musculaire).
To convert biomass as energy, one can consider that on average, organic matter (mainly C-H-O-N atoms) provides 18.5 Mega-Joules per kg of dry matter (MJ/kg). Further, on average carbon represents about half the mass of dry biomass. The chemical energy in the form of organic matter produced by photosynthesis is therefore of the order of 8.5×1021 Joules per year (8500 EJ; Figure 1). An initial yield of photosynthesis, with respect to the solar energy available at the surface, can be estimated as 8.5×1021 / (3×1024), i.e. about 0.3%. This yield can be refined by restricting the solar flux to the part which can be effectively used by plants: for example, deducing hot and cold deserts, or accounting only for the ‛active’ part of the solar flux, the red and blue wavelengths that are useful for photosynthetic reactions. The yields are then higher. This overall yield of 0.3% therefore corresponds to the capacity of the living world to exploit the solar energy available throughout the Earth.
The yield considered afterwards is lower by a factor of 2: in fact, to carry out this photosynthesis, a photosynthetic organism must maintain its cells, and also must grow and reproduce, all of which requires energy, taken from the photosynthesised biomass. Photosynthetic organisms thus breathe in about half of their biomass production. The rest is called the net primary production (Figure 1). It is measured by the increase in organic matter (mass per year). This is, for example, the growth of a forest estimated by the forester, or a crop productivity estimated by the farmer. An estimate of this overall NPP can be expressed as the mass of carbon stored per unit area per year (Figure 2).
A strong contrast in productivity exists between the continents, where productivity is generally limited by water and temperature, and the oceans, where it is limited by light and nutrients (Figure 2). Coastal waters are very productive due to the input of nutrients from the continents, but the centre of the oceans, the subtropical gyres [2] (in black on figure 2), are large deserts of productivity due to the absence of nutrients. Due to their large surface area (2/3 of the Earth’s surface), the oceanic biosphere fixes almost as much carbon as the terrestrial biosphere, about 50 and 60 GtC per year, respectively, even though marine biomass is much less exploited than terrestrial biomass.
The overall net primary productivity is thus of the order of 110 GtC per year, a figure that sets the upper limit for the sustainable use of biomass. This productivity provides a chemical energy stock of the order of 4.2×1021 Joules per year, or 100 Gtoe per year (Fig.1 and Table 1). The actual sustainable threshold for this use is not known, but is much lower than this limit, since this biomass is shared between all organisms that do not produce their own organic matter and depend, directly or indirectly, on photosynthesis (called heterotrophs [3]), including humans. These heterotrophs are in fact themselves necessary for plant productivity: some transform dead biomass into the nutrients needed by plants, others provide these nutrients to plants or even protect them, which is the role of mycorrhizal fungi [4]. These are fundamental roles of heterotrophs that have been replaced by the use of fertilisers, pesticides, and machines in the so-called ‛conventional agriculture’. The importance of ecological services provided by ecosystems to humanity is well recognized, and these services are at risk with the increasing pressure on ecosystems.
The question which matters is thus how much of this primary productivity is already used by humans.
2. What energy is extracted from this productivity to satisfy human needs?
Humankind uses this biomass to satisfy very different needs. Depending on the scope and type of use, estimates vary considerably.
Satisfying the most vital need, eating, requires about 30 ExaJoules or 0.7 Gtoe per year, in the form of chemical energy. What is the associated primary energy consumption? The Food and Agriculture Organisation of the United Nations (FAO) has the most comprehensive database and provides estimates of crop, livestock and fisheries production for most countries. It estimates that the amount of available food is, on average, 3,000 kcal per person per day worldwide, although there are of course considerable regional differences.
How much biomass is used to satisfy this primary need? The order of magnitude, around the year 2000, was 6 GtC/year, including crop plant products, fodder used by ruminants and phytoplankton used by fish. In energy terms, this biomass use represents 230 EJ per year, or 5 Gtoe, extracted from net primary productivity (Table 1). This order of magnitude was very close to the fossil fuel production at that time, i.e. 8 Gtoe! And seven times the vital energy need (0.7 Gtoe/year). Why such a difference?
Much of the agricultural and livestock production is lost or wasted. But the most important losses, notably the inedible parts of crops, such as stems, leaves, or others, have been deduced from the total of 6 GtC/year. Another more fundamental reason is that humans are omnivores, so that we occupy a fairly high place in the ‛trophic chain’. This chain describes the trophic relationships between living organisms (‛who eats whom’), and therefore also the energy flows between the ‛trophic levels’.
If all humans were strictly vegetarians, the vital energy requirement would be derived solely from plants, i.e. from the net primary production. In reality, human consumption is also made up of meat, fish, eggs and other products from animals, which can be herbivorous (which feed on plants) or carnivorous (which feed on other animals). This makes a huge difference because the energy transfer between two trophic levels is only about 10%: to produce 1 kg of meat, an ox has to graze ten times as much fodder (in energy terms). The reasons for this energy loss are the metabolism (general functioning, which consumes energy), and the peculiar digestion carried out by commensal micro-organisms.
The energy transfer from primary production to the trophic level TL is 1/(EF)TL-1 with EF the energy efficiency which is about 10%. Hence, if humans were strictly carnivorous, and ate only beef (herbivorous), then 10 times more energy would have to be drawn from primary production than with a strictly vegetarian diet. This would be even more so eating animals of higher trophic levels, predators, which themselves only eat animals (or, equivalently, beef fed on animal meal!). This is the case with large fishes: cod, for example, has a trophic level of 3 to 4 depending on its diet, implying a loss of energy by 100 to 1000 times from the primary production (marine phytoplankton).
On average, the trophic level of humans is estimated at 2.2. To understand the energy implication, we can first assume a diet composed of 80% plants and 20% beef. Assuming an efficiency of 10%, this 20% meat would require almost 3 times more energy to be drawn from primary production than if humans were strictly vegetarians (0.8×1 + 0.2×10 = 2.8). In reality, the energy efficiency of ruminants is less than 10%, and humans also consume fish (among other animals): humans therefore need to draw almost 5 times more from primary production than if we were strictly vegetarians, and ultimately 10 times more than our strict caloric requirement (Table 1).
GtC per year | EJ per year | Gtoe per year | |
Global net primary productivity (NPP) | 110 | 4200 | 100 |
Vital energy needs of humanity (6 billion people) | 0.59 | 23 | 0.55 |
Energy extracted from the PPN to ensure this vital need | 5.8 | 230 | 5.4 |
Share of NPP diverted to all human needs | 16 | 620 | 15 |
Primary fossil energy consumption (oil, coal, gas) | 9 | 350 | 8.3 |
Table 1. Net primary productivity (NPP) and its use by 6 billion people (around year 2000). NB: GtC = billion tonnes of carbon; EJ = 1E18 Joules; Gtoe = billion tonnes of oil equivalent (see Note #1 for conversion)
3. What sustainable use of primary productivity?
The world population is growing, with projections of 1 to 2 billion more people in 2050 than in 2020. The demand for food will therefore increase, as will the demand for energy, unless gains in energy efficiency compensate for the increase in energy demand. The International Energy Agency (IEA) predicts in its standard SPS scenario (based on the continuation of current policies until 2040), a 60% increase in the use of biomass for heating and a tripling of biofuels use for transportation, for a total of about 1 Gtoe (Read: Biomass supply chains key to develop bioenergy). Can we use even more biomass, replacing even more of the natural environment with crops and artificial forests? (Read: Combustion de la Biomasse et avenir de la forêt ? and Guyane : La biomasse-énergie en débat)
The use of primary productivity described above is only for vital energy needs (Read: Les besoins d’énergie). But primary productivity is used for many other vital purposes: fibers for clothing, wood fuel for heating and cooking (still in many countries), timber for construction. Using these natural structural materials, fibers and timber, avoids the energy consumption needed to manufacture their artificial equivalents, synthetic fibres, steel beams or other concrete. These uses of plants, which correspond to vital needs such as clothing, heating and shelter, require an additional 1 GtC of primary productivity for a total of 7 GtC per year.
Other uses of primary productivity exist and can be considered in this budget (Figure 3). All of these needs divert an even greater portion of natural primary productivity that could have been used by other living organisms. This is the concept of human appropriation of primary productivity. For example, forest fires destroy primary productivity. Infrastructure, cities, roads and so on, pre-empt areas that could be productive. Agriculture and agroforestry divert land from its natural production. This appropriation is estimated by reference to the potential primary productivity, which would exist without human activity.
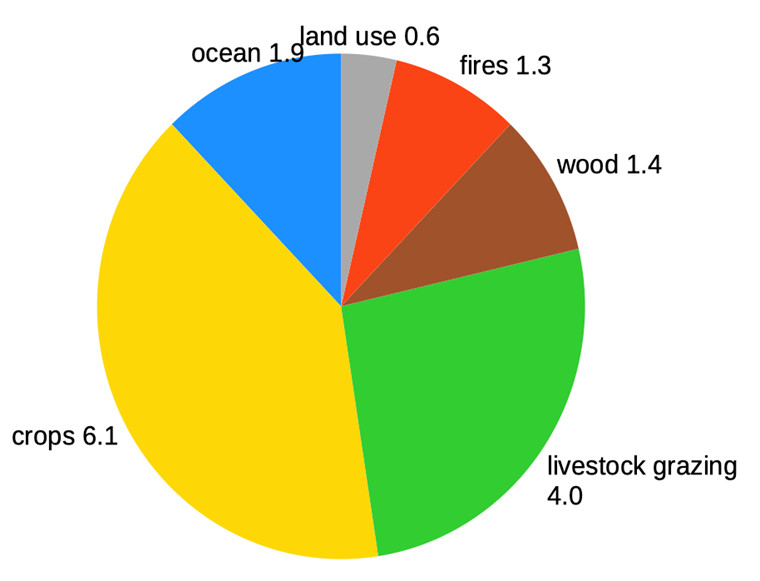
Figure 3: Human appropriation of net primary productivity around year 2000, expressed as energy flux (in Gtoe/yr, for a total of 15 Gtoe/yr, see Table 1 and note #1). The item ‛ocean’ represents sea products (fish, shellfish, algae, etc.). Note that an increasing part of fish production comes from inland aquaculture, fed with crop products. [Source : © G. Delaygue – UGA]
For the continental biosphere, this potential productivity is estimated at about 65 GtC per year, 10% higher than the estimated productivity (of 60 GtC/year). Human appropriation varies according to the definition used (and therefore the studies), and a value of 13 GtC per year can be retained for the terrestrial biosphere (Figure 4). This appropriation therefore represents more than 20% of terrestrial productivity. For the oceans, the appropriation is of the order of 2-3 GtC per year, or 5% of marine productivity. This use seems to be more limited, but the situation in the oceans is very different because oceanic primary productivity is consumed and recycled very quickly, on average in a few days, and is therefore no longer available (marine biomass, plankton, algae and fish, represents only 3 GtC of biomass, compared with almost 500 GtC of plants on the continents (Figure 4).
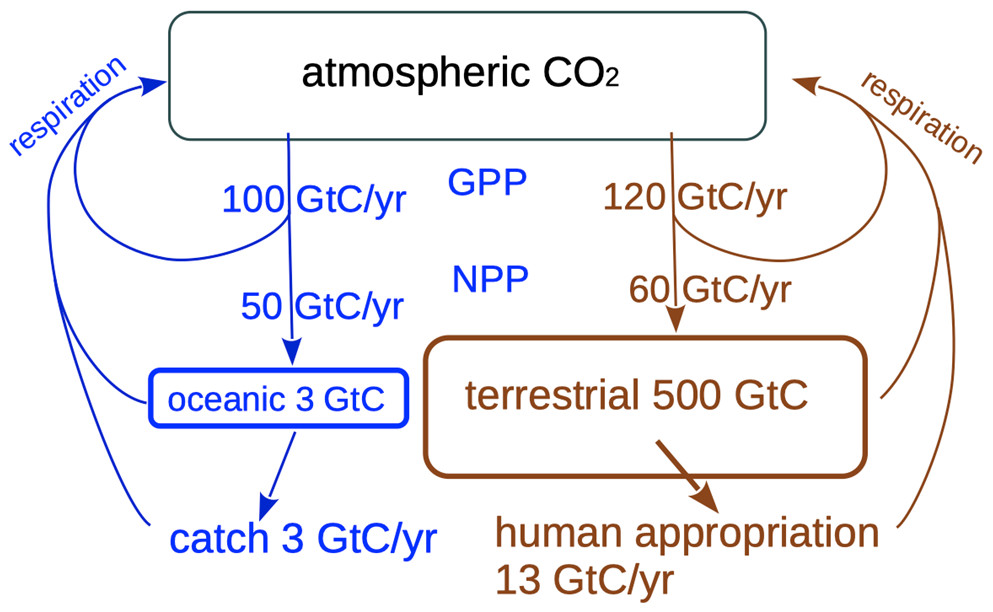
Figure 4: Fate of the net primary productivity in the global carbon cycle. The figure displays the carbon exchange within the biosphere, from atmospheric CO2 removal by photosynthesis (gross and net primary productivity – GPP and NPP) to CO2 release by respiration. Fluxes (in GtC/yr) are represented with arrows and living biomass stocks (in GtC) with boxes. Bottom fluxes correspond to human appropriation of PPN. [Source : © G. Delaygue – UGA]
Can this exploitation of the environment be increased further? Over the course of the 20th century, this exploitation increased much less quickly than the main indicators of human development, notably population. This decoupling is largely due to the enormous gains in agricultural productivity, which were made possible by the massive use of fossil fuels for fertilisers and agricultural machinery. The abandonment of these fossil fuels and the shift to biomass in the 21st century therefore poses a major problem, not only in terms of energy, but also in terms of the vitality of ecosystems and therefore the quality of the services they provide. It should be remembered, however, that some of the biomass used remains available in the form of plant waste (Read: Biomass and energy: from primary resources to final energy products).
But this energy confiscation has other environmental impacts, particularly on biological diversity: empirically, it has been observed that the diversity of an ecosystem, measured by the number of different genera, is directly related to its primary productivity. This confiscation is also accompanied, via agriculture in particular, by widespread environmental pollution and the extensive use of fresh water, which already limit primary productivity. Work carried out since the 1970s on the sustainability of this appropriation has shown the probable existence of thresholds of irreversible degradation of ecosystems, even if these thresholds are difficult to determine.
The regional view of this environmental pressure shows how critical it already is. The regional proportion of potential primary productivity appropriated by humans reveals this (Figure 5). In the most populated regions, diverted primary productivity was already at the same level as natural productivity, due to agriculture based on massive use of inputs and irrigation, and due to deforestation. This local production not only meets local demand but also, and sometimes mainly, that of regions far away, through globalised economic exchanges.
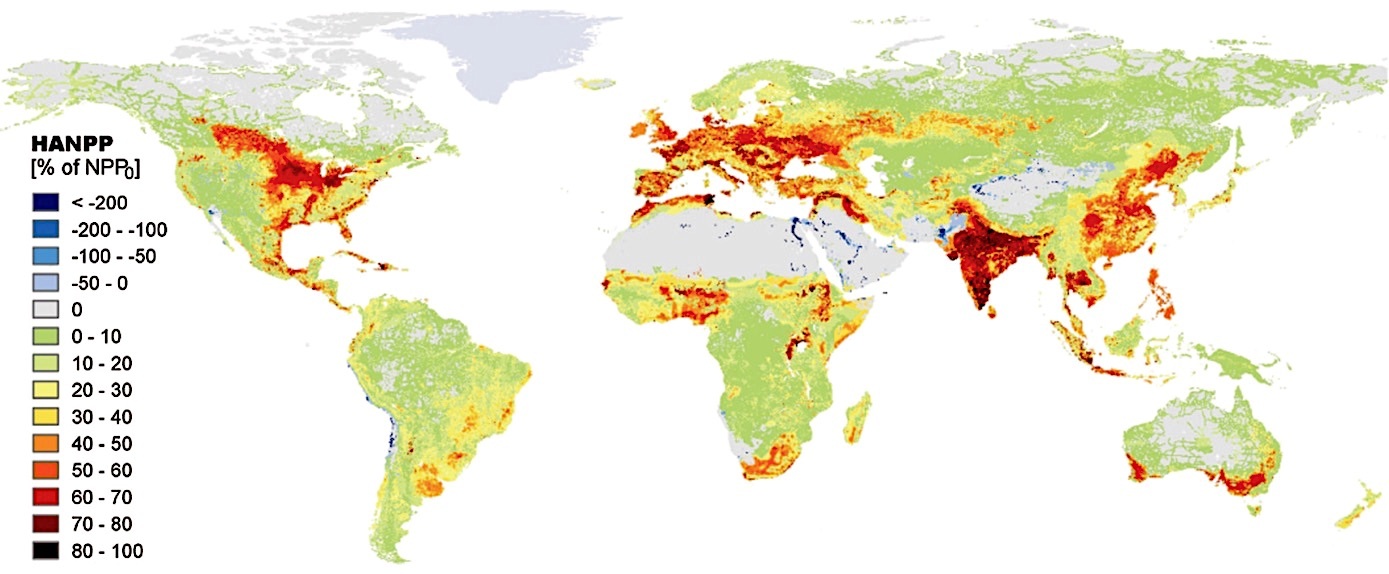
Figure 5: Regional (10 km scale) proportion of potential primary productivity appropriated for human needs, around year 2000 [Source: Haberl et al 2007]
Conclusion
The uses of biomass to meet vital human needs, such as food, clothing, heating and shelter, must be taken into account in humanity’s energy consumption, of which they represent a significant share. Not taking this energy share into account does not allow us to take full account of the coupling between energy and environmental issues.
Since the second half of the 19th century, the use of fossil fuels has allowed the world’s population and energy consumption to grow. A historical decoupling of this energy consumption from the use of natural biomass has taken place, thanks to the massive increase in agricultural productivity. The Earth has been transformed into a gigantic agricultural operation, at the expense of nature. This intensive exploitation raises many problems that were predicted decades ago and that have become unsustainable. Humanity’s energy needs will continue to increase: meeting them in a sustainable way, by abandoning fossil fuels, requires a massive improvement in energy efficiency. This improvement will be achieved in particular through diets: reducing the animal part of the diet will greatly reduce its energy, and therefore ecological, footprint. In addition, such a change in diet can also improve human health. For these two reasons, dietary change is one of the United Nations’ major recommendations for achieving the Sustainable Development Goals.
References
Cover image. [Source: Photosynthesis | Gardens of Mr. Khan, Paris. September 2016. | Lilac and Honey | Flickr]
Editorial (2022). The limits of biomass, One Earth. 10.1016/j.oneear.2022.01.001
Haberl, H. et al. (2007). Quantifying and mapping the human appropriation of net primary production in earth’s terrestrial ecosystems, Proceedings of the National Academy of Sciences. 10.1073/pnas.0704243104.
Krausmann, F. et al. (2013). Global human appropriation of net primary production doubled in the 20th century, Proceedings of the National Academy of Sciences. 10.1073/pnas.1211349110
Springmann, M. et al. (2018). Options for keeping the food system within environmental limits, Nature. 10.1038/s41586-018-0594-0
Vitousek, P. M. et al. (1986). Human Appropriation of the Products of Photosynthesis, BioScience. 10.2307/1310258
[1] Conversions: 1 calorie = 4.18 Joules; 1 Watt = 1 Joule/second; 1 ton of oil equivalent = 42 billion Joules (Read: Energy Units).
[2] Subtropical gyres: the largest oceanic regions between the equator and the tropics, characterized by an eddy-like circulation
[3] A heterotroph is a living organism that feeds on other organisms
[4] A mycorrhiza is a very close association between a fungus and a plant via its roots, each organism helping the other according to its respective capacities