No transition from electrical systems to renewable energy production with variable contribution (ENRv) without the development of storage facilities guaranteeing the balance and stability of the system, it is frequently thought. The reduction in costs of certain storage technologies, in particular lithium-ion batteries, would thus make the path towards self-production based on photovoltaic (PV) panels credible. However, are some difficulties not underestimated?
In developed countries engaged in renewable energy-based decarbonation policies, electricity storage is one of the priorities for action. This is the case in Germany with its Energiewende policy, which aims to develop up to 80% of renewable energy in the electricity sector by 2050, in particular by subsidising batteries associated with PV panels (Read: Figenschaften einer Stromversorgung mit intermittierendem Quellen). This is also the case in California, which wants to be at the forefront in decarbonizing its economy with a very ambitious policy of ENRvs in electricity production (50% in 2030), and which has set itself a target of 1,325 MWe of storage by utilities on their networks in 2025.
It is therefore an autonomous development of storage units “by the market” to meet the new needs of the electricity system for flexibility and security of supply services created by the deployment of ENRv. Built based on the income that an operator would find on different markets organised around electricity, such a development is not at all assured.
This is understood by remembering the different services that storage can provide: support for self-consumption, support for ENR production to make their production pilotable (dispatchable) as that of conventional techniques; contribution to the balancing and frequency adjustment reserve services required by the system manager; opportunities to save in peak capacities and network reinforcements whose needs are increased by the integration of ENRv at any point in the transmission and distribution network. These services depend for each installed unit on the chosen technique, the size of the unit and its technical characteristics (charging or discharging speed, efficiency).
Before presenting the various technical and economic functions of electrical storage (section 2), it is essential to examine the different storage methods, the state of their development, as well as their characteristics that determine the services they can provide to the various actors and the overall electrical system (section 1). This will be followed by questions on the economic possibilities for future developments of the various electrical storage solutions (section 3).
1. The different techniques of electrical storage
The introduction of different types of electrical energy storage (EES) technologies into the system is one of the main changes expected in the coming years, due to promising cost reductions, made possible by various learning experiences. In the more or less long term, the costs of storage techniques could fall to around 50 to 100 €/MWh discharged, which would put them at a good level (Figure 1). This evolution is due to the current shift of these technologies from a niche market to large-scale development in support of the development of renewables at various levels.
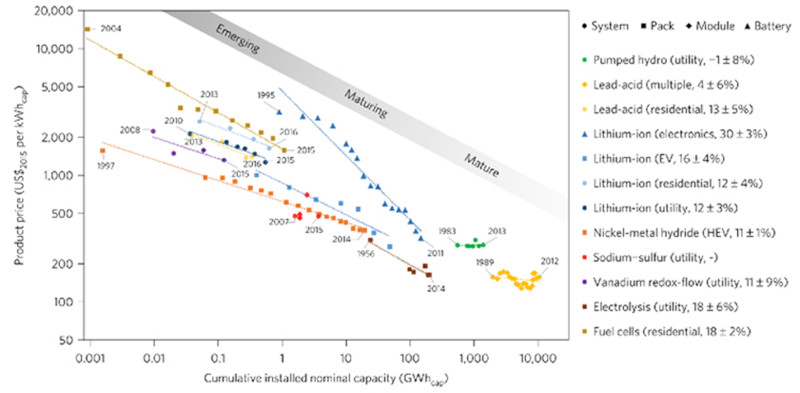
Fig. 1: Graph of cost reductions at each tenfold increase in installed capacity by storage technology. [Source: Schmidt et al, 2017]
Storage can be deployed at the level of the transmission network, distribution networks and consumers. It can offer several services to the overall system when it is managed entirely by the transmission system operator (TSO) with possibly the distribution system operators (DSOs) when storage facilities will be installed at this level thanks to smart grids since some of the intermittent ENRs are connected at this level. The same applies to the individual owner, when the storage is installed behind the meter and coupled with his own production by PV panels.
From a technical point of view, storage technologies can be classified according to the energy conversion process involved[1], namely:
- mechanical energy, including pumped hydraulic storage, compressed air energy storage and flywheels;
- electrochemical energy, consisting of batteries such as various types of Li-ion technologies, advanced lead-acid batteries, but also circulating batteries such as Vanadium-Redox and Zinc-Brome batteries (Read: Accumulators: the future of energy storage).
- hydrogen (H2) which involve technologies combining electrolyzers and fuel cells (FC); or, instead of fuel cells, a transformation of hydrogen into methane, which is then used in a gas-fired combined cycle power plant, the so-called “power to gas” which is supposed to allow inter-seasonal transfers of electrical energy (Read: Fuel cells).
- electromagnetic principles such as the storage of superconducting magnetic energy via supercapacitors (Read : Superconductivity and its applications).
The last two categories are far from economic viability, in particular the “power to gas” which fascinates many engineers by its utopia of a completely carbon-free electricity system from which nuclear power would have been banned and which would therefore be entirely based on renewable energies. It is only in such a system that inter-seasonal storage could have a useful purpose, but this would ignore a very low overall yield of 20-25%, and an economic balance sheet that will remain negative for a very long time with a very high cost per kWh returned.
On the other hand, the state of development of the first two categories deserves to be examined.
1.1. Storage based on transformation into mechanical energy
In the first category of storage by transformation into mechanical energy, pumped energy transfer stations (PETS) are the most mature storage technique. They are already commonly installed in electrical systems in developed countries (140 GWe installed worldwide, including 43 GWe in Europe and 4.2 GWe in France, the main plant being the Grand’ Maison WWTP with 1.6 GWe (Read: Pumping stations). Appropriate sites in France and Europe have become scarce, but a number of equipments with tanks could be re-equipped to become WWTPs at reasonable costs.
The storage of compressed air energy is less well known. It consists of compressing air by consuming electricity during off-peak hours. In the case of a system with a large share of ENRv, it is therefore a matter of storing energy in hours of excess ENRv production in the system and then expanding it in a turbine to restore part of the initial electricity in a discharge time ranging from a few minutes to two-three hours. Several techniques exist that differ from each other in the management of cooling and heating of the air during the compression and expansion phases. For example, the most advanced adiabatic technique consists in recovering the heat from the air after compression in a thermal storage to use this heat during the air expansion phase. Less expensive, diabatic storage does not include heat recovery and release techniques (Figure 2).
Storage takes place in geological cavities. Two compressed air storage units have been developed worldwide: one installed in the United States with a capacity of 110 MWe with a discharge time of 26 hours, the other in Germany in Huntorf with a capacity of 290 MWe with a discharge time of 3 hours.
The flywheel technique consists in storing energy in a rotating mass in kinetic form so that it can be used when the balance of the system requires it. Two experimental flywheels of 775 tonnes and 9 metres in diameter to supply 1 MWh at a capacity of 400 MWe in landfill on 15 minutes were tested during the 1980s, near Oxford. Recently, 200 flywheels with an individual capacity of 100 kW have been installed to provide frequency control services to the New York State electrical system.
1.2. Electrochemical storage
On the battery side, alongside traditional lead-acid batteries used in cars and alkaline batteries for electroportable use, emergency lighting and aeronautics, progress has been made in electrochemical storage units that are resistant to many cycles, with good efficiency and energy density: Sodium-Sulphide (NaS) batteries for stationary storage, metal-air batteries, lithium-ion batteries and REDOX circular batteries, the latter two techniques showing the greatest promise for electricity networks.
Lithium-Ion batteries, on which a large part of R&D has been concentrated, have the characteristics of a high energy and power density (10 times more than lead batteries), as well as an excellent efficiency of 90%. They also benefit from greater depth of discharge, but also from greater flexibility (no need for discharge and full recharge) with a limited impact on their life span. However, at the current stage, the technique still presents risks of thermal runaway. Industrial units stacking Li-ion batteries have begun to be built for networks integrating large ENRv capacities. A 20 MWe device was installed in the North-eastern US PJM system to provide frequency control service to the grid[2]. Tesla’s 100 MWe Li-ion battery unit was installed at a 300 MWe wind farm in South Australia in late 2017. In Great Britain, a 49 MWe project is currently being carried out on the National Grid in 2018.
On the other hand, REDOX circulation batteries (or flow batteries) have the particularity of storing the recharged energy in two electrolytes Zn+ and Br- contained in separate tanks. Electrochemical charging and discharging reactions take place by circulating chemicals in solution on the surface of the electrodes. There are many REDOX battery concepts, the most established being based on vanadium salts. This concept makes it possible to increase the energy capacity independently of the power capacity. The guaranteed life and number of cycles of these batteries are as important as those of Li-ion batteries, but their efficiency is lower. Projects are installed on King Island in Tasmania with an average battery capacity of 200 kW/4 hours or 400 kW/10” in peak periods and in Japan where, since 2005, a 4 MW/90′ unit has been associated with a wind farm while a 15 MW/60 MWh unit was built in 2015 by Sumitomo. In China, Dalian is currently being installed at 200 MWe/800 MWh and Primus Power at 25 MWe/75 MWh.
1.3. The determining characteristics of the services provided by a storage technique
The key characteristics of storage technologies that determine the economic services that can be provided to the power system, or to an investor-operator in liberalized power sectors, are the size, power to energy ratio, discharge and recharge rate, efficiency and number of charge-discharge cycles that they can withstand over their lifetime (aging). Short-term storage refers to small cycle periods ranging from a few seconds to a few hours, while long-term storage ranges from a few hours to several weeks (Table 1). The ability to follow a fast control signal for frequency regulation, which requires short ramp-up times, differs according to discharge speeds. The size of the installation also determines its placement in the system at the transmission or distribution network level, at the decentralized level or behind the meter of a self-producing consumer.
Technologies | Capacity | Minimum load in storage | Discharge time at nominal power | Efficiency | 2020 projected cost** (
€/kWh) |
Supercapacitor | 10kW-10MW | Seconds | nd | ||
Flywheel | 10kW-1MW | Seconds | 80% | 3500 | |
Batteries Li-ion | 1kW-1MW | 20% | Minutes (20’-2h) | 80-90% | 300-400 |
Batteries NaS | 15MW | 10% | Minutes (20’-2h.) | 80-90% | 330 |
Batteries REDOX flow* | 100kW-15MW | 10% | Hours (about 3h) | 70% | 1500 |
Compressed air energy staorage (CAES) | 10 -200 MW | 15% | Hours (about 5h) | 50% | 750 |
Pumped hydro storage (PHS) | 200-1000 MW | 10% | Hours (about 24h) | 75-80 % | .nd. |
* Vanadium redox flow batteries
** Sources on costs: Carlsson, 2014 ; Zerrhan et Schill, 2015 ; Zackaeri et Syri , 2015 ; Schidt et al., 2017. |
Table 1: Characteristics of the different electrical storage techniques.
Currently, the most mature and widespread storage technology is hydraulic pumping or PHS. Nevertheless, technologies such as Li-ion, REDOX flow and compressed air storage batteries have made significant technical progress, resulting in cost reductions per learning effect. Major progress in cost reduction is expected from the transition to very large-scale battery manufacturing and assembly plants (such as the “gigafactory” for manufacturing the Li-ion battery sets installed by Tesla and Panasonic in Nevada) to achieve significant cost reductions.
However, these potential cost reductions do not necessarily indicate that electricity storage techniques will spontaneously develop in the electricity sector with increasing shares of ENRv. The units to be installed must find an economic value for the services they can offer and, to this end, the organisation and regulation of the electricity sector concerned must enable investors to find sufficient income on service markets in which their storage unit participates, alongside the energy and capacity markets.
To understand this diversity of potential revenues, it is necessary to consider the different stages of an electricity market, including the hourly energy market, the balancing product markets, the system services market and above, the capacity market mechanism that pays for the guaranteed power (Figure 6 in Annex).
Technologies | Capacity
kW/MW |
Discharge time | System integration | Service | |||
Frequency setting | Network constraint resolution
(short term) |
Wholesale electricity system management
(Arbitration) |
Contribution to capacity
cutting-edge / network strengthening |
||||
Supercapacitor | 10 kW
-10 MW |
Secondes | Distribution network | + | + | ||
Flywheel | 10 kW-1 MW | Secondes | Distribution network | ||||
Batteries Li-ion | 1 kW
-1 MW |
Minutes/ hours | Individual & Distribution network | + | + | -/+ | |
Batteries NaS | 15 MW | Minutes/ hours. | Transmission & Distribution network | + | + | -/+ | |
Batteries redox flow | 100 kW- 15 MW | Hours | Transmission & Distribution network | + | + | -/+ | |
CAES | 10 MW-
200 MW |
Hours | Transmission network | + | + | ||
PHS | 200 MW-
1000 MW |
Hours (24h. and more) | Transmission network | + | + |
Table 2: Services provided by the different storage technologies according to the duration and speed of discharge.
Storage has the potential to offer several services, including: storing energy from solar installations in residential and commercial buildings, managing the timing of energy injections from wind or PV installations into the system pending higher prices (arbitrage), providing frequency regulation system services, improving the management of the transmission grid or distribution networks due to new congestion created by new wind turbines or PV farms, and contributing to security of supply at peak and during critical periods. The services that each storage technology can offer are provided on different temporary scales (Table 2). These services depend on the characteristics of each technique, while the size of the units determines their level of insertion into the electrical system (transmission system level, HV or MV levels in the distribution networks, or the level of autoproducers).
2. The different economic functions and services of electrical storage systems
These functions are as follows: support for the self-consumption of individual or community PV panel production; complementing decentralised electrification solutions in developing countries; arbitrage between the production and injection of ENRv production on the network and the services provided by storage to the system economy with the development of the supply of frequency control services (system service) to absorb the variability of ENR production, contribute to solving the network congestion problems they cause and contribute to long-term security of supply.
2.1. Facilitate the self-consumption of individual productions by PV panels
It is one of the first applications we think of. With the addition of battery storage for individual or commercial autoproducers equipped with photovoltaic panels, more of the individual PV production can be self-consumed rather than exported to the grid. This production is never in phase with the power demand of the autoproducer. The latter is necessarily connected to the grid to meet its complementary supply needs.
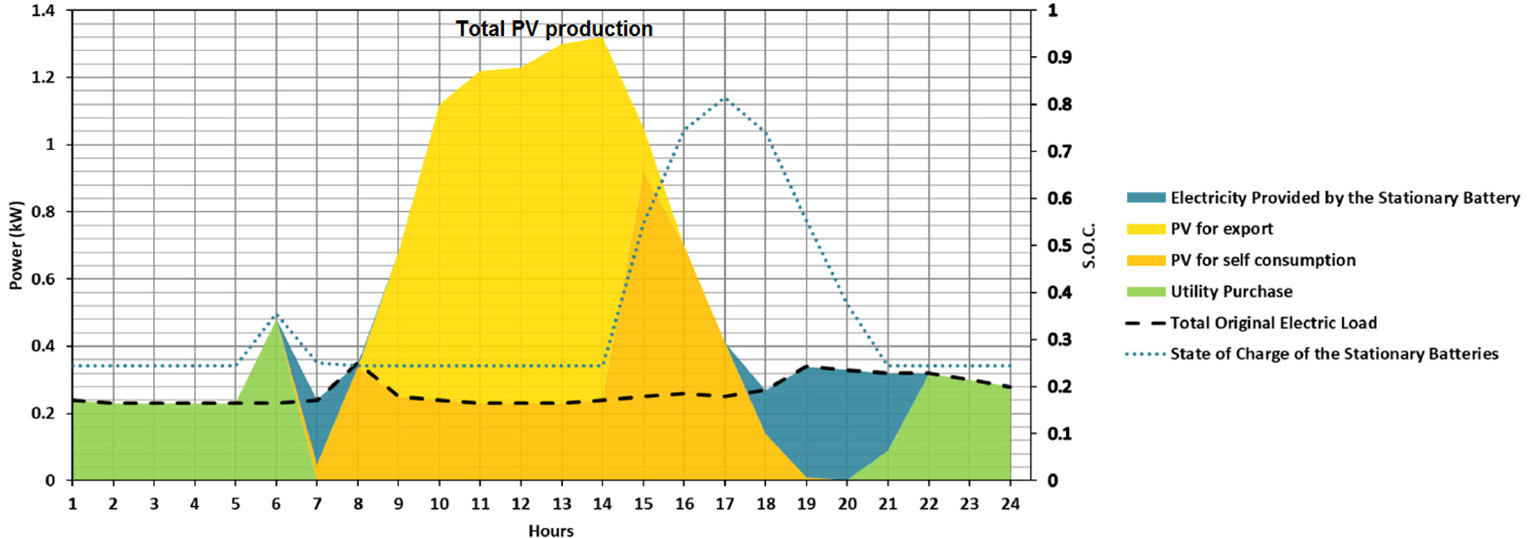
Fig. 3: Graph of the impact of individual storage on the daily self-consumption of a self-producer by PV. [Source: adapted from Sani Hassan et al, 2017]
With a battery sized to its production capacity, it can reduce its purchases from the network, the stored kWh that can be consumed later when PV production stops. Battery storage therefore improves the adequacy of production for individual consumption. The rate of self-consumption is high. In practice, the one associated with a 3 kW panel can increase from 30% to 50-60% in Germany with a 1 kW battery with a charging time of 5 hours (Figure 3).
The addition of batteries improves the economy of the PV installation by limiting electricity purchases from the grid to a high rate, this advantage can be increased if this rate is differentiated between peak hours (morning and evening when the sun disappears) and base hours during the day. The reduction in the overall costs of its supply is all the more important for the autoproducer as electricity storage takes place during hours when retail rates, more or less aligned with hourly wholesale market prices, are low, while the energy that has been stored is consumed when prices are higher, particularly during peak hours of the day.
However, if these properties of coupling PV equipment with batteries appear attractive for us to move towards the new world of decentralized production, which is much talked about, the economy for the overall system to which the autoproducers remain connected is absolutely not assured.
Of course, individual self-generation supplemented by a battery can be profitable for a private individual compared to the purchase of electricity at retail prices in countries where prices are high. When the cost of self-consumed energy, linked to the investment cost of PV panels, is lower than the electricity rate, we speak of grid parity. This is the case in Germany, where the model has been taking off since 2013-2015: self-production by PV panels has developed there with a system where only surplus production is remunerated at a feed-in tariff lower than retail prices including VAT. However, this is only an apparent competitiveness. The real reason is not mainly the decrease in the costs of PV panels and batteries, but the presence of high taxes and fees, the latter being used to finance the additional cost of promoting electric ENRs, which is quickly very high, particularly because of feed-in tariffs.
Taking the example of Germany, where the retail rate excluding tax is 110 €/MWh (including 50 €/MWh for energy) in 2016, the fee for ENR is 65 €/MWh (more expensive than the price of energy), while the other taxes are 75 €/MWh, reaching 250 €/MWh. To make a comparison, the feed-in tariff for PV electricity, more or less aligned with the cost price per MWh of an individual PV installation, was around €130/MWh.
Moreover, the overall economy of the electricity system is affected by this evolution because the tariff signals on the various services offered by the system to the self-producer are not “efficient”. Indeed, they do not reflect the real costs of the services provided to the self-producer, since the latter uses the supply of the network during hours when the energy of its PV panels, returned by the battery, is not sufficient to meet its needs.
While the distributor’s costs, which are essentially fixed infrastructure costs, remain unchanged despite the self-production of a growing number of connected customers, its revenues are falling, preventing it from covering its fixed costs. Indeed, the distributor’s revenues include revenues related to the subscription (the fixed part) and the “energy” part of the tariff, i.e. the part that depends on the quantity transported. Subscriptions alone are far from covering network costs. Here the self-producer does not pay the full cost of the distribution service. Network service rates are overwhelmingly based on energy, not on power, which is the guaranteed distribution and supply service offered to the self-propelled vehicle. Uncollected revenues are passed on to other consumers, including households with incomes well below those that can afford to finance the installation of their PV panels. In other words, the self-generator does not pay for the guarantee of supply service through the centralized system and the transmission and distribution networks necessary to carry the additional electricity when the self-generator needs it.
This difficulty leads to a fundamental problem of the development of decentralised storage and production of electricity by renewable energy sources supported by policies of subsidies for the PV system and batteries: still in Germany 40,000 units with batteries were subsidised in 2016 up to 500 €/kW of batteries up to 30 kW. The development of this system on a large scale not only jeopardises the overall economy of the system itself by leading self-producers not to pay for the services provided to them, but also has so-called “redistributive” effects to the detriment of small consumers who pay more for the distributor’s services than they should and who effectively finance part of the policy in favour of renewable energies. Since grid service tariffs are based on energy, an increase in the number of self-generators means that a larger proportion of grid costs will be passed on to consumers who are not able to finance the installation of PV panels and produce their own electricity. This problem is beginning to be put on the table, but it is difficult to explain and make people understand that because of the lack of “economic regulation” of the electricity system, the long-term global optimization of the electricity system and the “individual optimizations” do not converge.
Moreover, by imagining that self-consumers pay the right price for the service provided to them by the network, the development of pro-consumers (prosumers), i.e. self-producers-self-consumers equipped with batteries, has other shortcomings compared to the overall economy of the system in which they remain integrated. Indeed, while storage capacities are installed by prosumers, the overall system in which they are integrated does not really need them to manage the variability of all ENRv productions, including those of individual or collective autoproducers (energy communities). Individual storage batteries are not operated in the sense of balancing the overall system. In addition, the flexibility resource is by far not the cheapest to install, compared to other flexibility resources such as large battery units that would be installed on the grid at a cost three times lower per MWh, or erasure aggregator programs[3].
2.2. Storage as a complement to decentralized electrification solutions in developing countries
Support for the development of rural electricity supply in developing countries is the case where electrochemical storage can find its full economic significance for decentralised electrification. Extending networks is not always the best solution. Mini-grid or off-grid solutions based on individual electrification based on PV panel kits and batteries are less expensive and capital-intensive, which then struggle to find profitability with electrification through rural distribution networks (Read: Energy supply to off-grid populations in Africa: diagnosis and solutions).
The economy of mini-hydraulic and PV panel solutions is to be compared to that of a supply by generators powered by diesel or biofuels dependent on transport. These solutions, for the same service rendered, can be economical provided that they include a high storage capacity in relation to the power requirements, to allow a reliable supply, particularly during night hours and in the event of a decrease in solar or hydraulic gains.
On the other hand, household solvency can be a major obstacle to the solution of “PV + batteries” kits, but there are now electrical service offers in which the kits are provided by specialised companies that also provide maintenance, in return for a fairly low monthly rent payable by mobile phones. Thanks to these kits, customers can light up, recharge their phones and power a number of household appliances. More than 100,000 customers have been equipped in Tanzania and Kenya since 2011. In Côte d’Ivoire, Electricité de France (EDF) set up a joint venture called ZECI in 2016 with a Californian start-up OffGrid Electric to market this type of kit with the aim of serving two million Ivorians (500,000 households) within a few years. (Read: Rural electrification in sub-Saharan Africa).
2.3. The combination of storage with a PV or wind power plant for controllable delivery to the grid
By placing a storage unit near a wind or PV farm, this function consists in making its production controllable (dispatchable) by smoothing its delivery to the system. More specifically, in liberalised market systems, it consists in storing its energy produced in off-peak hours when prices are low, to deliver it to the grid and to the market during peak hours when prices are higher, which is made possible by a regulatory framework in which ENRv producers no longer have priority for injection into the system and are exposed to hourly market prices
The value of such an application in relation to the system must be put into perspective in developed economies with mature and well meshed power systems, as it is much more interesting to manage intermittency at the level of the whole system. At this level, in fact, we can benefit from the abundance of variable production and effectively organize the backing of all “flexible resources” to provide the service of “rapid reserves”, among which storage techniques are only one possible resource. The others are the use of demand cancellation programs set up by cancellation aggregators, very fast ramping combustion turbines[4], as well as the call for imports via interconnections.
When the problem posed by the intermittent production of a wind or PV unit is at the distribution grid level, the distribution grid operator (DSO) will also compare the use of different solutions to manage this problem: in the short term, capping of wind generation (against remuneration of the “capped” producer); in the long term, reinforcing lines or storage facilities. This solution tends to be the last resort in well meshed systems whose markets are liberalised because the principles of competition prevent network operators (DSOs, like TSOs) from deciding to install and manage storage units, as is the case in the European Union.
That said, the storage solution is of great interest in island areas with much less mesh network, reserve margin and expansion of ENR-variable production, and in the absence of interconnections with other systems. The use of these storage solutions makes it possible to develop ENR production on a larger scale. Thus, in the French Overseas Departments and Territories (DOM-TOM), two calls for tenders for ENR production included a storage constraint on developers defined by a smoothed profile of deliveries to the network or delivery delays during peak hours (Read: Storage of renewable energy in the form of hydrogen for isolated sites).
This solution is also of interest in the power systems of developing countries with a less meshed and less dense network than in developed countries. Storage reduces system costs resulting from intermittent production of large wind turbines and PV farms. It is interesting to make the production of large PV farms or wind farms that have recently been developed (2015) in many countries (India, Pakistan, South Africa, Morocco, Egypt, or others) more manageable under long-term contracts at guaranteed prices with public companies.
But cases can also be found in developed countries with a low-density network. In Australia, for example, a 100 MW/129 MWh Li-ion battery unit using Panasonic-Tesla technology was installed at the end of 2017 in conjunction with the 300 MWe wind farm in Horsndale, developed by NEOEN (Néo-énergies), a French-owned company. However, this installation was only made possible by the support of a long-term contract with the State of South Australia, which guarantees the income from the battery equipment.
2.4. Arbitrate between production and injection of ENRv productions on the network
In a logic similar to that of ENRv producers using battery storage, this function is clearly part of a financial logic. A medium-sized storage unit installed by an investor would allow MWh to be stored during low price hours and discharged during high price peak hours. It is a strategy of “trader” trading between the prices of successive hourly markets[5]. It could be that of a pumping station owner with arbitrations for the week, more difficult for the month. It all depends on the amount of energy that can be stored and the ratio of stored energy to power during discharge, which determines the discharge time.
It is also a strategy of a producer with several types of power plants, including ENRvs, that seeks to optimize its revenues: it uses the power generated by low-fuel-cost base plants during off-peak hours when prices are low, to return the MWh stored during peak hours; it avoids using its high-fuel-cost combustion turbines and limiting the number of starts that cause equipment fatigue and increase maintenance costs. The postponement of MWh injection is most interesting in systems with a high proportion of production by low-cost base stations (nuclear) or a high proportion of fatal production by ENRvs or run-of-river hydraulic equipment. This is an ideal function for WWTP pumping stations that pump water from their lower basin to their upper basin during off-peak hours of the day or week. These arbitrage strategies are “win-win” for the economic efficiency of the overall system since they can reduce operating and fuel costs by reducing the use of combustion turbines during peak hours.
However, the future revenues of a storage unit intended mainly for arbitrage are not easy to assess in advance in order to decide on the investment based on the expected revenues. If the differences between off-peak (night) and peak (day) prices are reduced due to the increase in photovoltaic generation in the system, due to the off-market feed-in tariff scheme, the profitability of the planned WWTPs may be so affected that the projects will be abandoned. This was the case in the 2000s for four projects in the Swiss Alps well connected to the German system, which were condemned because of the large PV capacity installed in Bavaria, which reduced the gap between day and night prices on the EPEX electricity market.
2.5. Services provided by storage to the electrical system
Storage provides services to the overall system economy with the development of frequency control services (system services) to absorb the variability of ENR production, by helping to solve the network congestion problems that these productions generate and by contributing to long-term security of supply, which is increased by the presence of these ENRv productions (Figure 4).
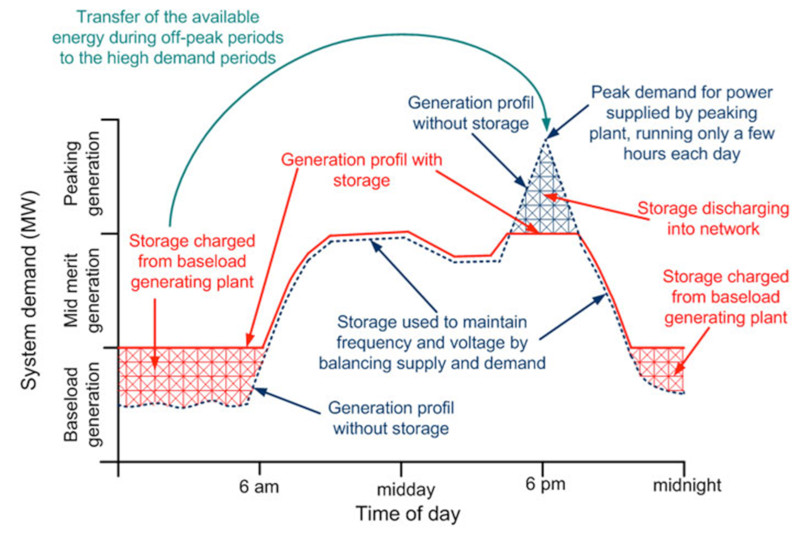
Fig. 4: Graphs of the services provided by the storage facilities to the electrical system at the T&D network level. [Source: ESA]
2.5.1. The offer of frequency control services
The development of storage facilities improves the system’s ability to absorb the variability of ENR production. Electricity grids experience continuous imbalances between electricity production and consumption, with millions of uncorrelated uses that are now increased by fluctuations in ENRv production. These imbalances cause the frequency of the electrical current to deviate from the 50 Hz standard, which can damage sensitive equipment. If they are not controlled, they can become too large and affect the stability of the network. Storage systems are particularly well suited for frequency control due to their fast response time and ability to charge and discharge quickly.
In high contribution ENRv systems, frequency control to ensure real-time supply-demand balance is made particularly complex. It requires the mobilization of different types of reserves with different dynamic characteristics. There are primary reserves (Frequency Containment Reserves) that act automatically in 30 seconds, the time to stabilize the frequency. There are also secondary reserves (Frequency Restoration Reserves) that also act automatically, but in a few minutes. And there are tertiary reserves (Replacement Reserves) that are manually activated in 15 to 30 minutes to replace previous reserves in order to maintain system reliability and deal with possible new incidents.
The use of system services (frequency control reserves) offered by production equipment is a cost for the operators of this equipment, as these are all MWe that are not used to produce and sell on the hourly market. The use of battery or compressed air storage services that can offer frequency control services, because of their fast response (less than a second for some techniques), avoids setting aside conventional production capacities, which can then be used to deliver energy at the right time and generate revenue from hourly energy markets in the best way.
For this to happen, the remuneration for frequency control services must be effective and adjusted accordingly. It is already different from one country to another. Sometimes, without payment as in Italy, services can be remunerated for an annual contract awarded by an auction system, which reveals the value of the reserve service as in Germany, France and the United Kingdom. There is also a remuneration by a performance payment for the speed of the response to the call of the system manager, as in some electrical systems in the United States. This payment method has already encouraged investment in the installation of about 100 MWe of batteries in US electrical systems.
What about the services offered by electric vehicle (EV) batteries?
Considering the different uses of battery storage invariably leads to the question of electric vehicles (EVs) and the possible participation of their batteries in the provision of system services to transmission or distribution system operators whose balancing task is complicated by the presence of significant ENRv production. The idea is attractive, but this possibility cannot compete with other means of providing such services to the system manager who planned the development.
On the other hand, controlling the management of EVs battery recharges appears to be a much more effective issue for network managers (Figure 5). During the charging phase of their batteries, EVs could provide a high degree of flexibility through several types of actions: starting the charge, stopping and restarting it, modulating the charging power, or delaying or postponing the charging process. It is typically a battery charge erasure service that EVs owners can offer through aggregators, each of which would manage several hundred contracts with EVs owners. This service can grow in size as the number of EVs grows. At the overall system level, it would save investment in flexible means of production, including combustion and gas turbines.
On the other hand, it is more difficult to demonstrate the value of the system services that could be offered by aggregators playing on the discharge of a large number of EVs batteries, or that could be directly sought by the grid operator. Studies have shown that this flexibility offered by EVs batteries is much less valuable than simple erasure actions on the power demand of manufacturers and consumers in the tertiary sector. However, the offer of system services by EVs batteries is an argument that EVs promoters frequently put forward to give an image of modernity and economic efficiency to the costly policies of deploying this type of vehicle. They can indeed highlight the ability of EVs to actively integrate into real-time electricity markets (balancing market and system services) and not only by complicating the management and development of electricity distribution networks. In fact, the deployment of EVs will first and foremost allow the development of a significant potential for load shedding alongside other flexibility resources, including the traditional potential for shedding in the industrial and commercial sectors. (Read: The car of the future: competing energy technologies).
2.5.2. The participation of storage in solving network congestion problems in systems with high ENRv contribution
The presence of storage facilities of various types in a system is used to facilitate the management of the transmission or distribution network, which is complicated by the presence of many ENRv units. The ability to install storage devices also opens up options in network investment planning. Storage can avoid (or delay) network reinforcement investments that would be required if large ENRv capacities were to be installed, resulting in the emergence of network constraints at congestion points. As the latter are problems located at specific points in the networks, storage facilities must be located as close as possible to the congestion points that we want to manage as effectively as possible.
However, the storage units to be installed are not the only solutions. They can compete with other means of flexibility such as quick-start turbines, consumption erasure, advanced inverters that allow fine management of frequency regulation, or the capping of disturbing wind or PV production when the regulations define precise rules governing this capping. These other options may be preferred to the installation of a storage unit when it comes to investing based on the revenue to be expected from the sale of system services and adjustment by the storage unit once installed.
In fact, the market context does not favour this kind of approach, unlike the old context in which network and production investments were the responsibility of the planner of the public service monopoly, a planner who could detect all the benefits of the storage unit for the network if European regulation of the electricity industries did not prohibit network operators from investing in and operating storage units for fear that they would distort the functioning of the time market. However, one could imagine that a network operator, foreseeing the gain of delaying a network investment by installing storage at a specific point in the network, could trigger an investment by an independent operator in a storage unit through a tender for a long-term guaranteed revenue contract that ensures the profitability of the investment. It remains to be seen whether the ultra-liberal rules of electricity regulation in the European Union will allow such an initiative in the future.
2.5.3. The contribution of new storage facilities to the adequacy of system capacity
The problem of ensuring security of supply in any situation is complicated by the development of large-scale ENR production, since “doldrums”[6] without wind or PV production could occur during peak winter hours in conjunction with an episode of extreme cold. In countries with strong development of electric heating such as France, this type of extreme cold episode has already encouraged investment in peak equipment (combustion or gas turbines) and erasure programmes, via capacity compensation mechanisms. This is the main motivation for the implementation of the decentralised capacity obligation mechanism, decided in France in 2017, which will therefore be reinforced in the future by the development of wind and PV capacities.
The installation of storage units that will contribute to the provision of system services can also prevent the construction of an additional peak power plant. These units therefore contribute to the security of supply of the system and as such should benefit from the additional remuneration offered by the capacity remuneration mechanism, which remunerates the MWe of plants whose availability is guaranteed during peak and critical periods. In some electrical systems in the United States, which also have a guaranteed capacity remuneration mechanism, battery units already benefit from it. Thus decisions to invest in storage units may be triggered by prospects for additional revenues, in addition to revenues in the system services and adjustment markets.
3. The economic constraints of the development of electrical storage
The reduction in the costs of the various storage techniques, particularly those of lithium-ion batteries, is not enough to ensure the shift, imagined by many analysts, from a centralised electrical “paradigm” to a decentralised paradigm based on “distributed” and individual production based on intermittent renewable sources. There are at least two reasons for this. First, because it is the economic value of the services that each new storage capacity of a particular technology will provide in the context of the system to which it belongs, in particular ENRv’s share in production, which determines the chances of its development. Secondly, because the markets for each flexibility service must be sufficiently developed to provide investors in storage units with income that corresponds to this economic value. If this were not the case, it would be necessary to rely on a planner who can decide on the optimization of the power system and the resulting investments to achieve the decarbonation objectives of the power system by using only ENRv, which is no longer really in the air.
3.1. Difficulties in assessing the chances of developing different storage techniques
Expectations of the development of electrical storage and the various techniques concerned are complex to establish because of the diversity of services provided by storage, but also because of the competition between storage and competing flexibility solutions, more traditional ones such as rapid ramp production units, or those requiring less capital such as demand cancellation programmes[7]. Implementation decisions are also complex to establish because the economic value of the services provided by a storage unit to be installed changes as a result of the own development of electrical storage through a kind of self-cannibalization. The more we do, the more the economic value of all installed storage capacities decreases.
A second problem is rather that of a hypothetical planner and no longer that of a potential investor who cannot recover all the benefits that his investment would bring to other producers and system operators: it consists in the fact that the economic value of new energy storage capacity for the system is linked to the structure of the existing system and its capacity and network congestion constraints. This economic value depends both on the existing technological mix, in particular the place of hydraulics and ENRv in production, and on the state of the transport network and its development possibilities. The latter can be blocked by opposition to the installation of transmission lines, while the increasing integration of wind or PV production is likely to cause congestion. In Germany, where most of the wind power capacity will be developed at sea or near the North Sea, the blocking of the construction of new very high voltage (VHV) and high voltage (HV) transmission lines will create many congestion points. As a result, simulation models of the German power system identify the value of installing 3000 MWe of battery storage near grid congestion points in northern German regions.
More generally, the level of interest in deploying storage depends very strongly on the systems in which it is carried out, with particularly marked differences between those with a well meshed network and those with a dispersed network frequently encountered in some American or Australian regions, emerging economies and developing countries. This interest also depends on the importance of the penetration of ENRv at the different levels of the system from centralised production and transmission through the distribution network to the final consumer. Finally, it depends on the pre-existence of hydraulic equipment, particularly reservoir power plants that offer great flexibility, as in the Scandinavian countries, Austria, Switzerland and the Canadian provinces.
A third difficulty in anticipating the economic interest of developing storage of different types is the gap between the economic value that storage capacities of given characteristics can have for the system[8] and that of a potential investor who could develop such storage capacities by anticipating revenues that he could recover on the various markets (energy, adjustment mechanism, system/reserve services, capacity) and that could cover his fixed and operating costs.
In other words, the planner who controls all investment and operating decisions and who could anticipate all the future benefits of installing new storage capacity at different levels of the system, could precisely decide to develop this capacity. On the other hand, potential investors in each of these capacities could not decide to invest in the majority of cases for at least two reasons:
- failure to take advantage of the benefits that its new installed capacity would bring to other actors (network operator, ENRv producers, among others) and,
- in the absence of being able to really anticipate the future benefits related to the interference that the decisions of other investors in production, but also in storage, would have with its future benefits; even the decisions of PV investors will have repercussions on its future earnings through arbitrations, as noted above.
In the end, even in this logic of individual decision-makers, which has predominated since the liberalization of electricity systems whose coordination is based on markets, it is possible to trigger investment in electricity storage units. First, by ensuring that the various economic services offered by storage equipment are remunerated on the electricity markets by improving energy and adjustment markets, and by developing system service markets (reserves to regulate frequency and voltage). Secondly, by providing them with additional remuneration through the legalization of the participation of storage units in the capacity remuneration mechanisms being set up in European countries.
Nevertheless, will these different sources of income be sufficient to trigger investments in storage units, taking into account the price and volume risks that will have to be taken? In case of doubt, other mechanisms should be available to ensure that investors receive income covering their fixed costs and an adequate return on their invested capital. Such schemes could be modelled on the auction for long-term guaranteed income contracts for wind turbine and PV farm projects, which the European Commission has forced Member States to adopt. On all these subjects, studies and, a fortiori, experiments are still lacking.
3.2. What about future developments in electrical storage?
There is nothing to prevent us from simulating the “optimal” evolution of the electrical system after a large-scale development of ENRvs has been imposed by 2030 and then 2050. This exercise should not be equated with the search for an optimal mix under the constraint of limiting CO2 emissions in a restrictive way, a particularly interesting research in France where the electricity mix is practically carbon-free as it is in Sweden, Norway, Switzerland, British Columbia or Quebec[9]. However, it is possible to imagine an ideal regulation by a planner seeking to optimize the electrical system over time by deciding to install storage units complementary to those of the ENRvs. The decision to develop them would be linked to the value of the services provided to a system supposedly gradually invaded by ENRvs thanks to the closure of nuclear power plants imposed by the government.
In this logic, an “order-based” optimization model would compare the costs associated with the installation and operation of each storage unit with the benefits that their services bring to the electrical system, i. e:
- limiting capital expenditure (networks, peak and flexible power plants),
- the reduction of operating costs by participating in daily or weekly arbitrations of the system subject to “forced” injections of MWh from wind farm or PV production,
- the reduction of operating costs by providing frequency control services, the need for which is significantly increased by the development of wind power and especially PV,
- maintaining security of supply and limiting carbon emissions by limiting the use of combustion turbines.
However, in general, when comparing the economic interest of the different storage techniques and on the basis of their current costs, it can be seen that:
- it is the STEP pumping stations that are competitive for sites that can still be equipped or dams to be built as STEPs;
- this is followed by the storage of batteries (10-100 MWe) in large units in the transmission network and in some of the distribution networks that are congested with ENRv production, particularly when the installation of new lines is subject to strong social acceptability constraints;
- as for the individual storage associated with individual production per PV panel, it is two to three times more expensive than storage units installed specifically near congestion points in the transmission or distribution networks; PV+ batteries are developing, because the prices of the actual services that the network provides to each autoproducer/consumer are generally very undervalued and because autoconsumers do not pay the cost of the ENRv promotion policy and the taxes for the kWh they consume, unlike other consumers. After rectification, the self-producer’s business model would have much less chance of being profitable and developing.
In addition, the general development of electricity storage will depend on competition from other means of flexibility, in particular demand cancellation programmes, fast ramp combustion turbines, but also imports of electricity and system services through interconnections, which are likely to be developed. It is therefore necessary to consider all these elements at the same time and not to consider electrical storage as the necessary guarantee for the development of large-scale intermittent production in electrical systems.
Annex
The different stages of the market design of an electricity market.
In theory, a series of markets ranging from futures markets (hourly energy futures contracts) to real-time markets (balancing markets), day-ahead markets and intraday markets are required to establish a price or value on the various attributes of the electricity product (energy, supply guarantee, product quality (frequency, voltage). Price signals from energy markets (day ahead markets, intraday markets) are not sufficient to provide the right investment incentives to market players, making it difficult to pursue the objective of maintaining security of supply during critical periods. Nor are they sufficient to cover all the operational decisions that not only allow the system to be balanced shortly before the delivery time, but also guarantee the quality of electricity: maintenance of the nominal frequency and voltage, despite fluctuations in demand and intermittent production by the ENRv (Read: The complexity of electricity markets: the limits of the liberalisation of the electricity industries).
There is concern that the current electricity market provisions do not provide adequate investment incentives to maintain security of supply. Most of the electricity markets in Europe were energy only markets “only energy”. It is therefore necessary to add a specific capacity market mechanism to give income to the guaranteed capacities available during the critical hours of the year
The development of intermittent renewable energies reinforces the need to reward operational flexibility and the provision of system services at very short notice, both for flexible power plants, aggregator demand cancellation programmes and storage units. The value of operating flexibility is generally captured by price variations in intraday markets and should also be captured by system service markets that do not exist or that exist in too basic a way in different countries. There is therefore concern that short-term price signals may not reflect the scarcity of operational flexibility in many countries. A review of the current arrangements for intraday trade, balancing products for the TSO and for purchases of system services by the TSO are therefore under way.
Figure 6 illustrates an ideal market design linking the different market sequences several years in advance in real time, including capacity “markets”, day ahead and then intraday energy markets, balancing services and primary and secondary and tertiary reserves.
Additional bibliography
ADEME, ATEE, DGIS, Artelys (with G2ELab & Enea Consultant), (2013) Study on the energy storage potential Study and Synthesis. October 2013. Available by link: http://atee.fr/sites/default/files/peps_-_rapport_public1.pdf
EDF R&D, 2017. Electricity storage A challenge for the energy transition Paris: Editions Lavoisier. June 2017
IEA-ETP (International Energy Agency-Energy Technology Platform), 2015. Technology Roadmap. Energy storage 2015. Paris: OECD-IEA e.Library. https://www.iea.org/publications/freepublications/publication/TechnologyRoadmapEnergystorage.pdf
IFPEN, 2016. Current storage technologies and their state of maturity. Panorama 2013 sheet. Accessible through the link http://www.ifpenergiesnouvelles.fr/Espace-Decouverte/Les-cles-pour-comprendre/Le-stockage-massif-de-l-energie/Les-technologies-actuelles-de-stockage-et-leur-etat-de-maturite
Luo, X., Wang, J., Dooner, M., Clarke, J., 2015. Overview of current development in electrical energy storage technologies and the application potential in power system operation. Applied Energy 137, p.511-536. doi:10.1016/j.apenergy.2014.09.081
World Energy Council, 2016. E-storage: Shifting from cost to value in wind and solar applications. London: WEC Technical Documents. Available through the link https://www.worldenergy.org/wp-content/uploads/2016/03/Resources-E-storage-report-2016.02.04.pdf
Notes and references
[1] Luo, X., Wang, J., Dooner, M., Clarke, J., 2015. Overview of current development in electrical energy storage technologies and the application potential in power system operation. Applied Energy 137, p.511-536. doi:10.1016/j.apenergy.2014.09.081
[2] PJM stands for Pennsylvania New Jersey Maryland Interconnection LLC.
[3] In the electricity industry, an aggregator is an intermediary who aggregates flexibility services purchased contractually from consumers, such as centrally controlled load shedding on the devices of many small consumers, or a few large industrial establishments, etc.). An aggregator can also group together services-systems contracted with decentralized producers (cogeneration, wind turbines, etc.) as in “virtual power plant” projects. These services are then sold by these operators either on the adjustment mechanism or on the reserve markets where they exist. These intermediaries make it possible to scale up these services to the scale of what can be marketed in these stages of the electricity market.
There will also be aggregators to group and sell the energy productions of small wind producers in countries where producers are remunerated by selling on the hourly markets and then benefiting from additional remuneration, instead of selling at long-term defined feed-in tariffs.
[4] The ramp-up speed of a fast ramp turbine can reach 50% of its load after one minute and full power after two minutes, while a conventional turbine with the fastest ramp-up (e. g. General electric 7F-5, Siemens SGT6-500F) only reaches 30% of its load after 7 minutes and only reaches full power in 30 minutes. See https://www.wartsila.com/energy/learning-center/technical-comparisons/combustion-engine-vs-gas-turbine-ramp-rate
[5] It must be borne in mind that electricity is not a storable good such as oil or any other “convenience”, so markets have been structured into hourly markets for energy, and specialized markets for the various services that production equipment can offer to the system (rising and falling power offered for grid balance, system services such as frequency control and reactive power services (the annex explains this conception of electricity markets, known as “market design”).
[6] Borrowed from maritime terminology, the doldrums are an unstable weather zone characterized, among other things, by nil or changing and unpredictable winds.
[7] Villavicencio M. 2017 Analyzing the optimal development of electricity storage in electricity markets with high variable renewable energy shares. Doctoral thesis defended at Paris Dauphine, December 14, 2017.
Villavicencio M. 2018, The economic value of electrical storage in a system with a large share of ENR. Revue de l’énergie, n° 638, May-June 2018.
[8] What a hypothetical planner would master in deciding all investment and system management decisions but which no longer exists with the liberalization of industries.
[9] In other words, the large-scale development of ENRvs is generally carried out without any economic consideration. The target of 40% in 2030, 70% in 2040 and 90% in 2050 is totally arbitrary. The economist can or must comply with these prescriptions to try to remain useful…as we do here.