Before considering the role of nuclear power in the future electricity mix, it is important to know the origins of the industry. From the chance discovery of “uranium rays” at the beginning of the 20th century to the operation of a worldwide fleet of 450 reactors, the road is short but very rich.
At the end of the 19th century, a majority of scientists thought that there was not much left to discover in the field of physics. ” The atom did not exist”: some academics even said so.
1. Scientific discoveries
The history of nuclear energy began by chance on March 1896. For some time, Henri Becquerel, at the suggestion of Henri Poincaré, had been studying the fluorescence of certain bodies. He placed a photographic plate wrapped in black paper next to a salt of uranium sulphate that was supposed to fluoresce, i.e. emit X-rays when exposed to sunlight. In short, the uranium sulfate exposed to the sun becomes charged with light energy and is then able to give it back in the form of electromagnetic waves in the X-ray range. On that day, March1, 1896, Becquerel discovered that the blackening of the photographic plate occurred even when the salt was not exposed to sunlight. It therefore does not fluoresce. Uranium and its compounds emit specific rays by themselves. We will call them “uranium rays” for the time being (Read: Nucléaire : rayonnements, radioactivité et radioprotection).
From then, each year brought its share of discoveries:
– 1899: Ernest Rutherford identifies three types of radiation that he calls alpha, beta and gamma ;
– 1900: Marie Curie, born Maria Sklodowska, discovers another body with the same properties of emitting these rays; it is radium; she evaluates its atomic mass approximately and invents the word radioactivity ;
– with her husband Pierre Curie and Henri Becquerel, she received two Nobel Prizes: in 1903 for having discovered natural radioactivity, and in 1911 for having determined the atomic mass of radium (Figure 1);
– 1913: Niels Bohr applied his quantum theory to the hydrogen atom.
From 1913 to 1928, the Copenhagen School, led by Niels Bohr, laid the foundations of quantum mechanics. Paul Dirac, Wolfgang Pauli, Erwin Schrödinger and Werner Heisenberg were instrumental in establishing this theory, understanding the atom and explaining radioactivity. In 1932, James Chadwick established the existence of the neutron. The nucleus of atoms took on its current form as an assembly of protons and neutrons around which electrons gravitate.Starting in 1934, the neutron, because of its electrical neutrality, became the projectile of choice for bombing all types of elements. In particular, the astute Enrico Fermi, in Rome, bombarded with neutrons the last known natural element of the Mendeleev table, and therefore the heaviest, uranium, hoping to find new bodies, transuranics, but he came up with a perfectly incomprehensible result.It was not until 1938 that he began to unravel the truth and understand these experiments of bombarding uranium with neutrons. That summer, Irène Curie and Frédéric Joliot discovered, among the products formed in the bombarded uranium, a body with a period of 3.5 hours that seemed to have all the chemical properties of lanthanum. But what would it be doing there? They were very close to the truth but did not dare to publish it. In December of the same year, Otto Hahn discovered barium in bombed uranium. He then dared to assert that the nucleus had broken up. He discovered fission, which generated an extraordinary amount of energy.The following year, in 1939, Frédéric Joliot, Hans Halban and Lew Kowarski, at the Collège de France, demonstrated, in addition to the fission products and a large amount of energy, the production of two or three high-energy neutrons in uranium fission. They imagined the possibility of a chain reaction and took out three fundamental patents on behalf of the Centre national de la recherche scientifique (CNRS). These patents, which are very complete, can still be consulted at the Commissariat à l’énergie atomique (CEA). They provide all the information needed to build a nuclear reactor.
2. Military applications
These patents were never respected by the United States, which, with the outbreak of the Second World War, became the masters of nuclear energy research and exploitation.
On August 2, 1939, at the request of Leo Szilard and Eugene Wigner, physicists of Hungarian origin who feared that Nazi Germany would acquire an atomic bomb, Albert Einstein wrote to President Roosevelt to draw his attention to the new source of energy represented by uranium and to the possibility of building a bomb. From October 1939 to June 1942, the facilities needed to build an atomic weapon were quickly put in place: the Manhattan Project led by General Leslie Richard Groves and physicist Robert Oppenheimer.
The United States put in the resources. Three parallel isotope separation projects were launched in the summer of 1942. The gaseous diffusion method, finally judged to be the most promising, was industrially developed at the Oak Ridge center in Tennessee, where construction of the plant began in late 1942.
At the same time, on December 2, 1942, Enrico Fermi diverged the first graphite-moderated natural uranium atomic pile. This took place under the stands of the Chicago stadium, from which the famous photo has been preserved, bringing together, near the graphite pile, the cream of American physics at the time: Enrico Fermi, Eugen Wigner, Arthur Compton and a few others, while on the top of the stack a man is ready to slice with an axe the rope that will free the safety bars just in case… All these physicists, in fact, are totally unaware of the speed at which the divergence will develop because they know that the average lifetime of a generation of neutrons is of the order of one tenth of a millisecond. With only these so-called fast neutrons, the reaction can only get out of control and lead to a dangerous flash. Fortunately, nature has done things well: in each generation of neutrons, so-called delayed neutrons allow the reaction to be controlled. Enrico Fermi more or less knew this, but he was not sure of himself and took precautions (Figure 3).
A new step was taken in March 1943 with the arrival at Los Alamos of Robert Oppenheimer and a group of physicists charged with carrying out research on the criticality of uranium and plutonium, the latter being supplied by the enormous graphite batteries built in record time at Hanford.The results of this work were the development of a first bomb tested at Alamogordo (Trinity Test) and then of two other bombs, Little Boy in enriched uranium and Fat Manin plutonium, dropped over Japan. Although not very glorious, this act nevertheless contributed to stopping the war, and probably to preventing a third world conflict.In 1944, French physicists were non gratacin the United States. A team made up of Bertrand Goldschmidt, Jules Guéron, Hans Halban and Pierre Auger working at Chalk River, in Canada, nevertheless benefited from indiscretions that kept them informed of American work. She informed General de Gaulle of this during his visit to Ottawa in September 1944.Having fully understood the importance and necessity of developing nuclear energy in France, after the Liberation, he created the Atomic Energy Commission (CEA) on October 18, 1945. On December 15, 1948, at 12:12 p.m., the ZOÉ (for Zero Energy) battery diverged for the first time.
3. Electricity production
In December 1951, the Americans produced the first kWh of nuclear energy at the Idaho Falls site with the EBR1 reactor, which enabled them to light the small town of Arco in the State of Idaho. Over the next four years, development efforts intensified in the United States, the Soviet Union, the United Kingdom and France to move towards civil nuclear power. The first prototype power reactor was commissioned in Shippingport, near Pittsburgh, Pennsylvania, in 1957. It is a 60 MWe Pressurized Water Reactor (PWR).
But what is a nuclear reactor (Read: Les réacteurs nucléaires ; Les réacteurs de recherche ; Small modular reactors)?
An atom is made up of a nucleus of protons and neutrons, around which electrons gravitate. The binding energy of the elementary particles inside the nucleus is a few million times higher than the energy that binds the electron to its nucleus. This observation allows us to understand why we can extract a few million times more energy from the fission of an atomic nucleus than from the simple chemical reaction of this atom during combustion. In other words, one gram of uranium can produce as much energy as several tons of carbon products (coal, oil or wood).
How do we obtain this gram of uranium? Natural uranium is essentially made up of U 238 (99.3%), which is non-fissile and therefore does not enter the nuclear fission reaction, and only 0.7% of fissile U235. Only the latter is capable of providing fission reactions. To carry out fission, slow neutrons, known as thermal neutrons (2 to 3 km/sec) are needed, whereas the neutrons generated by the fission of the previous generation are fast (20,000 km/sec). It is therefore necessary to slow them down (Read: Le cycle du combustible nucléaire).
Light materials such as hydrogen, deuterium (an isotope of hydrogen) or graphite are good moderators. The neutrons, by successive shocks on the atomic nuclei of these materials, will thus slow down and be likely to create new fissions of the U 235 nuclei. Graphite, which does not capture neutrons, has the advantage of being cheap and allowing the use of natural uranium. Deuterium, in the form of heavy water, also has a very low probability of neutron capture, while allowing the use of natural uranium. The problem is that heavy water has a very high cost. It is produced by fractional distillation of ordinary water, which contains a very small proportion of heavy water. Hydrogen in the form of ordinary water slows down neutrons very well, but unfortunately also captures them. It is therefore necessary to enrich the uranium to the level of 3% U 235 to maintain the fission reaction.
When France, in 1957, considered using nuclear energy, it had no other choice than graphite as a moderator because it allowed the use of natural uranium. It is the natural uranium graphite gas (UNGG) line cooled with pressurized carbon dioxide, in parallel with the test of the heavy water gas line with the Brennilis prototype in Brittany. Great Britain also chose graphite-gas with its MAGNOX line, while Canada opted for heavy water in the framework of its CANDU programme. The United States, for its part, took advantage of the existence of enrichment plants to develop light water lines, PWR and Boiler Power Reactor (BWR), which allowed for more compact reactors that could be scaled up to very high power levels.
However, since Enrico Fermi, all physicists know that the future lies with fast neutron reactors, in which neutron slowing is avoided. These reactors are capable of converting 99.3% of U 238 (fertile) into plutonium 239 (fissile). The Pu 239, previously considered as a troublesome waste product, becomes a useful fuel. The amount of energy that can be extracted from natural uranium is thus multiplied by a factor of 50 or more. Under these conditions, the spectre of a nuclear fuel shortage disappears.
4. The French nuclear program
From 1963 to 1972, Électricité de France (EDF) started up six UNGG reactors. These were the first generation reactors: Chinon 1, 2 and 3, then Saint-Laurent 1 and 2, and finally Bugey 1, whose power peaked at 500 MWe. A final reactor of this type will be sold to Spain and built at Vandellos near Hospitalet del Infant in the province of Tarragona.
Since natural uranium is involved, the specific power of this type of reactor can only be small, i.e. 30 W/cm3 of fuel, and therefore the volume of the reactor is large, i.e. a cylinder 10 m high and 10 m in diameter, pierced by 4,000 vertical channels loaded with fuel elements cooled by carbon dioxide at a pressure of 25 bars. To contain this pressure, a three-metre thick pre-stressed concrete casing is required. In short: the size of a cathedral.
These reactors produce 1,500 MW of thermal power, which is transmitted via heat exchangers to a water-steam circuit expanded in a turbine linked to an alternator. This transformation of heat into mechanical and then electrical energy follows the Carnot principle. Given the temperature of the hot source (around 400°C), the efficiency of the installation is 30%. Only 500 MWe is therefore recovered. Two thirds, i.e. 1000 MWth go to the cold source, the Loire, the Rhône or the atmosphere with the use of air coolers. Considered not very scalable to large powers and providing a kWh at a high cost, this method was abandoned in 1969 in favour of pressurised water reactors (PWR) of the PWR and BWR type. These PWRs are known as second generation reactors.
Initially, two industrial groups proposed to develop boiling water reactors (BWR) and pressurized water reactors (PWR). It is this second solution that will be entrusted to the company Framatome, holder of the patent of the American company Westinghouse. The PWR has a compact core in the form of a cylinder 3 to 4 m high and 3 m in diameter contained in a steel vessel. It has some 40,000 rods of 1 cm diameter between which water at 150 bar pressure circulates, heating from 280 °C to 320 °C. The specific power of the fuel is 800 W/cm3. This water, from the so-called primary circuit, then passes through heat exchangers, steam generators or secondary circuit. The steam is expanded in a turbine coupled to an alternator. Once again, yields of 30% are required. A 1000 MWe reactor actually produces 3000 MWth.
With the 1973 oil crisis, under the presidency of Georges Pompidou, Prime Minister Pierre Messmer decided to launch a vast PWR programme, the project management of which was entrusted to EDF with the technical support of the CEA, the project management being entrusted to the company Framatome. From 1977 to 1995, EDF commissioned 58 PWRs, from 900 to 1350 MWe, with a total installed capacity of 63,000 MWe (Figure 4).
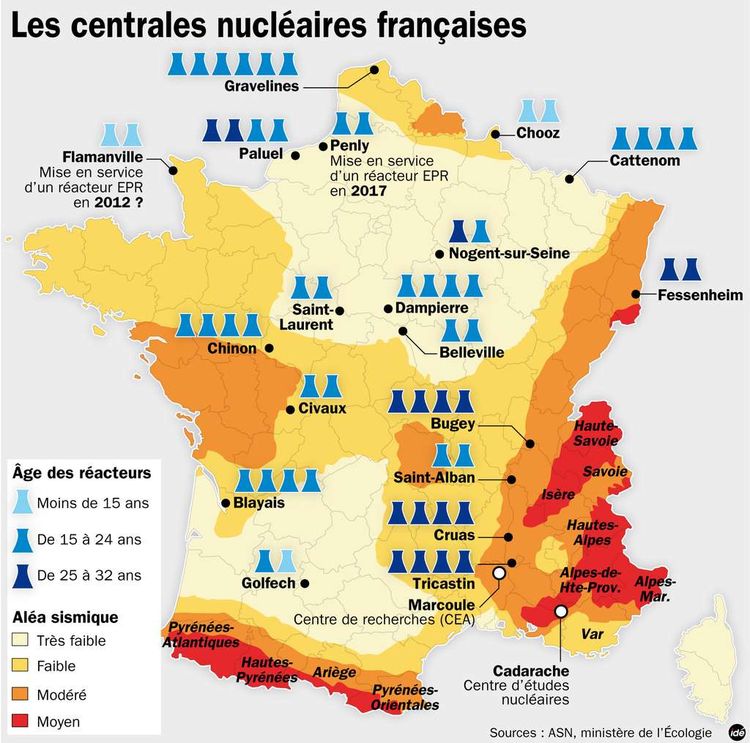
Fig. 4: Nuclear reactors in France. [Source: Sting and Roulex_45 and Domaina and Calvin411, CC BY 2.5 <https://creativecommons.org/licenses/by/2.5>, via Wikimedia Commons]
In 2017, France was second only to the United States in terms of the number of reactors and installed capacity, but it was well ahead in terms of the proportion of nuclear power in electricity production, at 72% (See: Énergie nucléaire : Les enjeux économiques and Le coût de production de l’électricité d’origine nucléaire en France). After the interruption of any new construction since 1995, EDF started in 2006 the construction of a 1600 MWe European Pressurized Reactor (EPR) in Flamanville, on the west coast of the Cotentin peninsula, which should be commissioned in 2015 and become the leading third generation reactor whose much improved safety would make a large-scale accident unlikely. A series of industrial difficulties has continuously delayed its commissioning, now scheduled for 2020.
5. Nuclear programmes around the world
As of 2016, 447 nuclear reactors are in operation worldwide, with a total capacity of 390 GWe spread over 20 countries on four continents. The countries with the largest nuclear power capacity are, in order, the United States, France, Japan and Russia.
Country
|
Number of reactors
|
Nuclear capacity
(Gwe)
|
Share of nuclear power
(%)
|
France
Slovakia
Belgium
Ukraine
Hungary
Sweden
Switzerland
Czech Republic
South Korea
Japan
Finland
Germany
Spain
United States
Taiwan
Russia
United Kingdom
Canada
India
China
World
|
58
4
7
15
4
10
5
6
21
50
4
17
8
104
6
32
19
18
20
14
439 |
63,1
1,8
5,9
13,1
1,9
9,3
3,3
3,7
18,7
44,1
2,7
20,5
7,5
101,2
5,0
22,7
10,1
12,6
4,4
11,1
374
|
76
52
51
48
42
38
38
33
32
29
28
27
20
20
19
17
16
15
3
2
13
|
Source: CEA. Elecnuc. Ed. 2017.
From 1957 to 1979, the United Statesbuilt 104 PWR and BWR reactors, representing an installed capacity of just over 100 GWe, the largest in the world, even though it accounts for only 20% of the country’s total electrical capacity. On 28 March 1979, the Three Miles Island accident shattered the dream of considerably increasing this share (see: Retour d’expérience sur les accidents nucléaires). Although this accident had no radiological consequences on the environment, the government decided on a moratorium on the construction of new reactors. In 2013, a recovery began but was halted by the fall in natural gas prices caused by the development of shale gas.
Poor in fossil energy sources, Japan relied early on the development of nuclear power to meet its rapidly growing electricity needs. At the beginning of 2011, 50 BWR and PWR reactors were providing more than 40 GWe of power. The Fukushima disaster, caused by an exceptionally large tsunami on March 11 of this year, halted the use of this energy source, whose future is now uncertain, as evidenced by its low contribution to electricity production in 2016.
In 2016, Russia had 35 reactors with a total capacity of 26 GWe. These are mostly VVERs, similar to Western PWRs, as well as RBMKs (enriched uranium, graphite-moderated and light water-cooled reactors). Under certain conditions, the latter are subject to operating instabilities that led to the Chernobyl accident in 1986 (see: Nucléaire : rayonnements, radioactivité et radioprotection). Western countries had studied this type of reactor in the 1960s, but, knowing the risk of instability, had never considered building it.
The rest of the world’s nuclear power belongs to :
– Germany, whose 17 reactors will soon cease to operate since, under pressure from the Greens, the country has decided to abandon all activity in this sector from 2022 ;
– the United Kingdom, which developed graphite-gas reactors as early as 1959, from which it obtained an output of around 10 GWe, but which was halted by the discovery of oil and gas in the North Sea until the recent resumption of activity, which has resulted in several PWR projects and possibly an EPR;
– Canada, various other European countries and emerging Asian countries (South Korea, China, Taiwan, India), which do not seem ready to give up nuclear energy, given their great need for electricity and the scarcity of other energy sources.
In 2017, the World Nuclear Association (WNA) recorded an installed capacity of 392 GWe from 352 active reactors (many of which are no longer in operation, particularly in Japan), representing a production of 2,506 TWh. Its 2050 targets are 1,000 GWe, or 25% of the global electricity mix.
Further reading
-Commissariat à l’énergie atomique (1998). 100 Years of Nuclear Power.
– Regards sur l’actualité. Le nucléaire français : Quel avenir ? La documentation française.
– Bonin Bernard (2012). Le nucléaire expliqué par des physiciens. Preface by Etienne Klein.
EDP sciences.
The Encyclopedia of Energy is published by the Association des Encyclopédies de l’Environnement et de l’Énergie(www.a3e.fr), contractually linked to Grenoble Alpes University and Grenoble INP, and sponsored by the Academy of Sciences.
To cite this article, please mention the author’s name, the title of the article and its URL on the Encyclopedia of Energy website.
The articles in the Encyclopedia of Energy are licensed under the terms of the Creative Commons Attribution-Noncommercial-No
Derivative Works 4.0 International License.