In the context of a transition to less carbon-intensive energy systems, hydropower is in the lead because it is renewable, flexible, storable and controllable. But do all these qualities have a downside? Identifying the environmental and societal impacts of hydropower allows us to prevent some and mitigate others.
With a share of around 60% of the world’s renewable electricity production, hydropower is the leading renewable source of electricity. It is also considered as a clean energy insofar as its exploitation does not produce any waste. However, several projects have been, or still are, the subject of strong criticism. Indeed, like any human activity, hydroelectricity is not without impacts on the environment and the riparian populations.
The great diversity of hydropower projects (Read: Hydropower: diversity and specificities) leads to a great variability in the nature and extent of societal and environmental impacts. The vast majority of them are linked to changes in the hydrological regime of the river, both upstream (creation of the reservoir) and downstream (flow programmes linked to production requirements). The more the hydrological regime is modified by the development and its operation, the greater the environmental impacts. It is therefore important that they be identified and assessed as early as possible during the identification and definition phases of new projects so that the relevance of the project is confirmed and its negative consequences are minimized or compensated.
1. Phases in the life cycle of a facility
The analysis of the clean and renewable character of a mode of electricity production must be carried out over the entire life cycle of the installations. The life cycle consists of three main phases: construction of the facilities, their operation, which is the period during which the facilities produce electricity, and finally, their dismantling.
For hydropower, as for all other electricity production methods, the construction and dismantling phases cannot be considered as clean and renewable. Sometimes very greedy in concrete or materials (riprap, sand, clay, among others) and made up of large equipment (turbine, generators, gates, or control equipment), the construction of the works requires the mobilization of natural resources which will not necessarily be returned to nature, even in the event of dismantling of the installations. Even though strict standards now govern construction sites in order to avoid discharging polluting effluents during construction, the large volumes of energy required, particularly for transporting equipment and earth-moving and construction machines, are the source of polluting discharges.
In the event of dismantling a facility, the same effects can be expected, but they are mitigated by the long service life of hydraulic facilities. Even after a century of operation, some dismantling is still not envisaged. When they are, it is most often,
- either during modernisation works, sometimes to reduce certain negative effects on the environment, or to improve productivity (Read: Rehabilitation of hydroelectric power plants) ;
- or as part of the restoration of the ecological continuity of a watercourse, as on certain tributaries of the Columbia River in the United States or, in France, on the dams of Vézins and la Roche qui boit on the Sélune (work in progress), Maisons Rouges on the Vienne, Saint-Etienne du Vigan on the Allier or Kernansquillec on the Léguer.
Even if this work represents only a very small part of the life cycle of major facilities, it obviously has similar impacts on their scale in terms of discharges or the use of non-renewable resources.
In the operating phase, a hydroelectric installation in good maintenance condition can be considered clean and renewable insofar as it does not consume any natural resources or produce any polluting waste. However, there may be discharges due to operating incidents, mainly discharges of petroleum products following accidental leaks of oil or grease, or discharges due to the loss of grease used to lubricate the dam gates. Today, accidental releases are eliminated by the use of technologies that do not require such products: Kaplan turbines equipped with oil-free hub runners, hydrostatic bearings and self-lubricating materials; biodegradable greases used to lubricate the operating elements of dam gates.
In total, hydroelectricity can be compared to other electricity production methods using the criteria adopted by the Interuniversity Research Centre for the Life Cycle of Products, Processes and Services (CIRAIG) of Polytechnique Montréal [1], namely:
- destruction of the ozone layer by quantifying emissions of ozone-depleting substances;
- acidification by quantification of discharges of substances contributing to the acidification of soil, groundwater or surface water, ecosystems and organisms;
- eutrophication by quantification of discharges of nitrogenous or phosphate materials contributing to the acceleration of the natural phenomenon of eutrophication
- human toxicity by quantifying discharges of substances with toxic effects on the human environment;
- photochemical oxidation by quantifying substances that are harmful to human health, ecosystems and crops;
- extraction of mineral resources by quantifying the mineral resources consumed;
- use of fossil fuels.
On the basis of the average values observed, with considerable variability for certain sectors, hydroelectricity
- is in first place for criteria 1 (almost no effect), 2 (almost no effect), 3 (almost no effect on average but great variability depending on the project), 5 (almost no effect),
- and second place for the others, with very low effects.
2. Greenhouse gas emissions (GHG)
Since the mid-1990s, the issue of GHG emissions from large reservoirs has fuelled controversy over the merits of hydropower development in the fight against climate change.
The construction of hydroelectric facilities and their eventual dismantling emit GHGs: concrete production, construction of underground structures by tunnel boring machine[2], use of machinery powered by combustion engines, among others. However, these emissions remain marginal given the lifespan of the facilities.
In the operational phase, hydropower was long considered to have no GHG emissions, but since the 1990s, studies conducted in Canada and then in the United States and Brazil have shown that large reservoirs can be a source of emissions. Since then, the challenges of global warming have prompted numerous studies aimed at assessing GHG emissions from natural environments (forests, rivers or lakes) and artificial reservoirs at different latitudes.
Whether they come from reservoirs intended for electricity production or for other uses such as irrigation or flood management, GHG emissions must be evaluated in net values, i.e. as a differential compared to the situation before the reservoir was created. In the current state of knowledge, it can be noted that:
- the net GHG balance of natural ecosystems varies considerably according to the type of environment, but also according to the period of the vegetative cycle (growth, maturity, decomposition) and climatic disturbances; to be meaningful, a balance should take into account a long period, of the order of a century, according to certain recommendations;
- even if the distribution of carbon between the soil and the vegetation varies according to latitude, and if they can be GHG emitters at certain times, forests remain carbon sinks overall, whether they are located in boreal or tropical regions;
- natural lakes and rivers are generally sources of GHGs [3]; their emissions depend on the input of the catchment area (and therefore on its characterization: nature of the soil, vegetation cover), on the depth, on the oxygenation rate, and on the living organisms they shelter (bacteria, plankton). Emissions are generally higher in tropical environment.
Older artificial reservoirs are similar, in operation and balance, to natural lakes (Figure 1). However, during the first few years following the impoundment of a reservoir, the submergence of vegetation and soils leads to high methane emissions [4]. In boreal regions, these additional emissions gradually decrease over time, and after about 10 years, the reservoir behaves like a natural lake. In tropical regions, these emissions may persist for longer periods.
As with natural lakes, the greater the surface area flooded and the shallower the depth, the greater the emissions from a reservoir, since a greater depth favours the oxidation of methane as it rises to the surface. Emissions are divided between emissions at the surface of the reservoir and downstream by the degassing of turbined water, which is taken from deep water and therefore has high methane levels.
From one study to another, the results are extremely variable because the GHG emissions are specific to each project: for Hydro Québec’s power plants, they are 6 gCO2 eq/kWh (run-of-river) and 17 gCO2 eq/kWh (reservoirs) [5]. In the case of facilities with particularly unfavourable characteristics (large flooded area relative to installed capacity, shallow depth, tropical zone, recent impoundment), the gross values are similar to, or even higher than, the emissions of thermal power plants[6]. On the other hand, some developments in tropical areas appear to be carbon sinks [7]: on average, compared to other means of production, hydropower generates very little GHG. In total (Figure 2), a comparison of the comparative emissions of the various existing or developing modes of production, over their life cycle and in gross value (without deduction of emissions before development), is very favourable to hydropower[8].
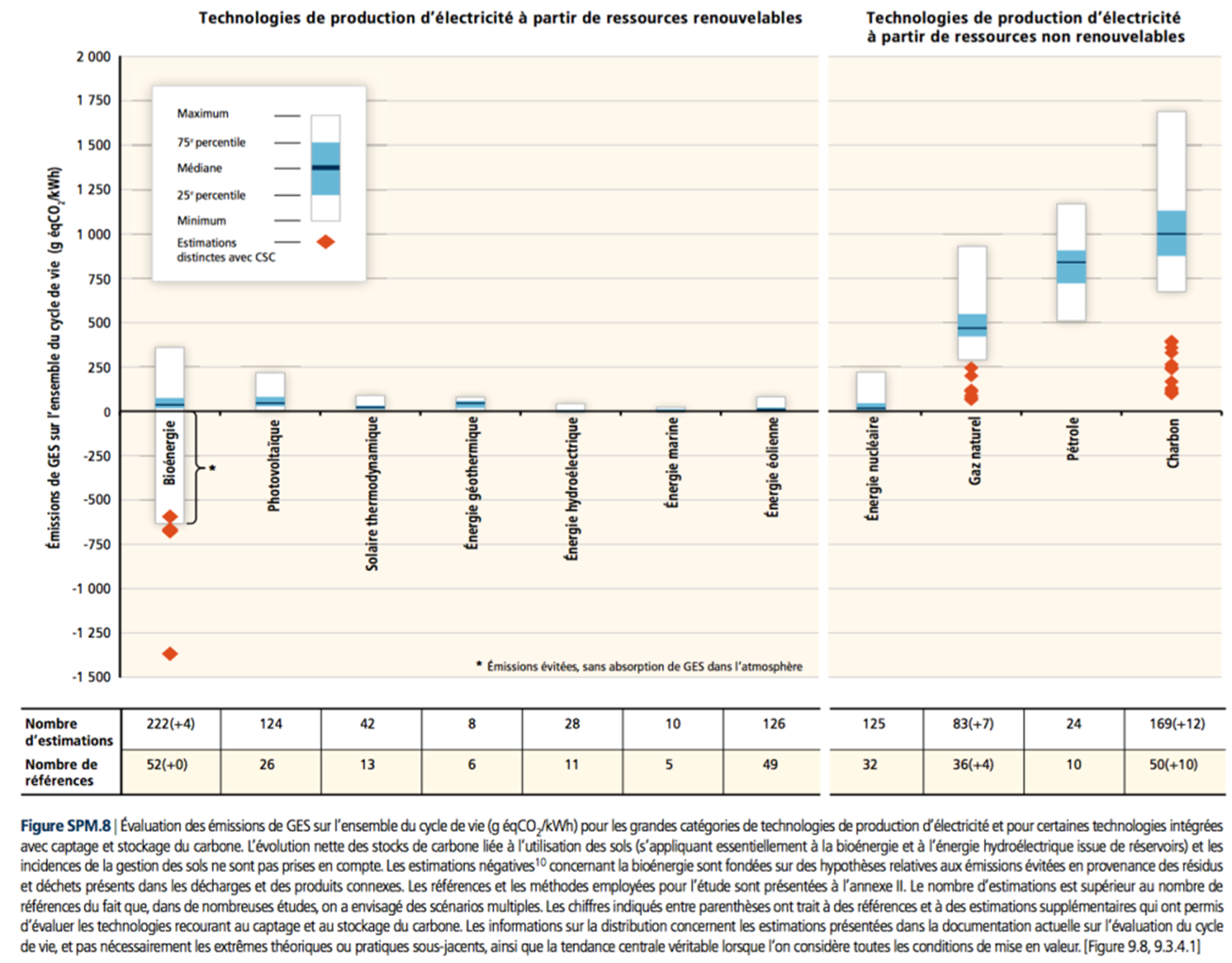
Fig. 2: Comparison of life cycle GHG emissions of different electricity generation modes. [Source: IPCC – 2011 – Special Report Renewable Energy Sources and Climate Change Mitigation]
The complexity of the biochemical and physical phenomena involved and the high variability of emissions as a function of many parameters do not currently allow for reliable modelling of the operation of a development from a GHG emissions perspective. The determination of the net emissions of a development therefore remains uncertain. Nevertheless, the design of a development in a tropical region should systematically aim at minimizing the flooded surface. At least until technologies for collecting methane from tropical reservoirs become available, it is proposed[9]. Used as fuel for gas power plants, the collected methane would reduce the reservoir’s impact on the climate and generate additional electricity production, estimated at between 15 and 55% depending on the developments studied.
3. Sediment transit
A river is not only water that flows, it is also solid materials: sand, sediment, pebbles, among others, which is called sediment transport. Depending mainly on the flow speed of the river and the granulometry of the materials, we distinguish:
- transport by scavenging, which concerns the heaviest materials, which roll along the bottom at a speed lower than the flow speed
- transport by suspension which concerns the lightest materials which are transported in suspension at the speed of the current.
A river in its natural state is the result of a balance between transported and deposited materials, a balance that evolves over the seasons according to the variations in the river’s flow. The construction of a structure in the bed of the watercourse disturbs this balance. Indeed, the modification of the sections of flow and thus of the flow speeds, involves a modification of the sediment transport. In the case of a dam with the creation of a reservoir, the sediment transport is completely interrupted, with multiple consequences.
3.1. Upstream consequences
Upstream of the dam, the flow velocity progressively decreases from the upstream end of the impoundment to the point immediately upstream of the dam, where the velocity can be considered as zero. The coarser materials are deposited upstream of the reservoir, followed by finer materials towards the dam (Figure 3).
This accumulation of material results in:
- the decrease in the useful capacity of the reservoir progressively reduces the modulation capacity of electricity production and, if necessary, the useful capacity of other uses such as irrigation or flood control;
- the raising of water lines upstream, in particular during flood episodes, which may lead to the flooding of regions normally not affected by the flood;
- in the long term, when the accumulation reaches the level of the water intakes, the entrainment of high concentrations of sediments leading to significant erosion of the intake structures (galleries, penstocks) and turbines; at this stage, the high concentrations released into the downstream river may have harmful consequences for the survival of the aquatic fauna;
- the storage of toxic substances (pollutants from the catchment area) in the sediments, which can be released during flushing or emptying operations.
3.2. Downstream consequences
Due to the blockage of sediments in the reservoir, there is a deficit of materials downstream, which results in the incision of the river bed, which may be accompanied by bank erosion. In the most serious cases, the deficit of transported sediments is felt as far as the mouth of the river and results in coastal erosion (regression of the coastline).
The modification of the hydrological regime, which is influenced by the management of the reservoir, also plays a role in the morphological balance of the watercourse, generally leading to an impoverishment, a standardization of the environments and an impact on biodiversity.
3.3. Case of diversion schemes
In the particular case of diversion schemes, the short-circuited section, due to the reduction in flow, loses its capacity to carry away the sediments brought by the tributaries, leading to the formation of alluvial cones in the bed of the watercourse. During floods, these alluvial fans obstruct the flow of the flood, which increases the risk of upstream flooding.
3.4. Sediment continuity
In order to avoid the negative consequences of the works on the sediment transport, several measures must be considered, on the one hand during the design of the development, and on the other hand throughout the operation, with the objective of ensuring the sediment transport in an environment-friendly way.
Preliminary studies for the development of a river for electricity production must include a hydro-morphological component to determine the volume of input, the sediment production zones and to characterise their modes of transport in order to take them into account in the location and capacity of future dams. At the level of each development, structures must be planned to allow sediment transport: settling basins with flushing and diversion structures, gates at different heights of the reservoir allowing controlled flushing, in particular.
Thereafter, operation must take into account periods of sediment supply (flood periods on the concerned river or its tributaries) and adapt continuously to retain as little sediment as possible. The volumes accumulated in the reservoirs can be regularly evacuated by flushing (Figure 4), possibly supplemented by dredging. In all cases, these operations must be environmentally monitored in order to respect acceptable concentrations for fauna and avoid the release of polluted sediments. A long-term economic vision is generally consistent with environmental preservation.
Fig. 4: Principle of the flushing carried out at the Génissiat dam. [Source [1].]
4. Geological and hydrogeological effects
The creation of reservoirs and diversion canals or the modification of the level of watercourses are not without effects on the level of the water tables. When the latter become troublesome by drying up wells or, on the contrary, flooding by raising the water table, measures are taken to counteract these consequences: creation of “contre-canal” (small canal parallel to the main canal) or lifting stations to replenish the water table.
Some earthquakes could be caused by the creation of a large reservoir[10]. Many microseisms have been well identified, but the most striking example would be the 2008 earthquake in Sichuan (China), of magnitude 7.9, caused by the impoundment of the Zipingpu dam. While microseismicity was indeed well recognized after filling, the causal relationship for the major earthquake remains debated as the impoundment could also have been an accelerating effect of an earthquake that would have occurred even without the impoundment.
5. Consequences for wildlife
The construction of facilities is not without effects on wildlife, both during construction and during operation.
5.1. When the reservoir is impounded
During the impoundment of large reservoirs, the gradual submergence of the land creates progressively submerged islands, leading to the drowning of animal species that become trapped there. As early as the 1960s, animal rescue operations, of varying degrees of success, were organized when the reservoirs were impounded. The ability to survive the disruption of their territory varies according to the species, and the release of the rescued individuals can create a local imbalance in the species, at least temporarily.
Insofar as the monitoring of these operations and of the individuals released in the years following impoundment has not always been sufficient, the value of these operations is still being debated, but they are often the occasion for a considerable enrichment of scientific knowledge and the discovery of new species. The best option for preserving fauna, however, remains the limitation of flooded areas.
The effects of dam construction on fish fauna are highly variable, depending on many parameters: the existence of endemic species, migratory species, the hydrological regime of the river, the characteristics of the reservoir and the type of operation, among others. The modification of the hydrological regime with the creation of an impoundment in place of a free-flowing river necessarily leads to a modification of the species represented, both upstream (in the impoundment and upstream of it) and downstream, and generally to a reduction in biodiversity.
5.2. In operation
The management of the development induces, downstream, variations in flow that differ from the natural hydrological regime. If these flow variations are not controlled, they can have negative consequences such as the destruction of spawning grounds by a rapid increase in flow during the reproduction period or the trapping of individuals during a decrease in flow.
Environmentally sound management must be based on operating instructions established on the basis of the results of site-specific environmental studies. They must take into account all the species present, the morphology of the watercourse and its environmental context, and make it possible to define, for each period of the year, the minimum and maximum flow values as well as the flow gradients authorised downstream of the installations and the minimum flow values to be ensured in the short-circuited sections.
In all cases, the downstream flow must respect a minimum flow value. The same applies to the short-circuited sections of the diversion schemes. This minimum flow, also known as instream flow or ecological flow, must respect the values that may be imposed by legislation,[11] but it should ideally be the result of a minimum biological flow study making it possible to define, for each period of the year, a minimum flow value to be respected.
5.3. Fish continuity
There are about 120 species of migratory fish: holobiotic species whose reproduction and growth zones in the watercourse are distinct and separate; amphibiotic species including anadromous species, living in the sea but reproducing in freshwater, which is the case of salmon; or catadromous species, living in rivers but reproducing at sea, which is the case of eels.
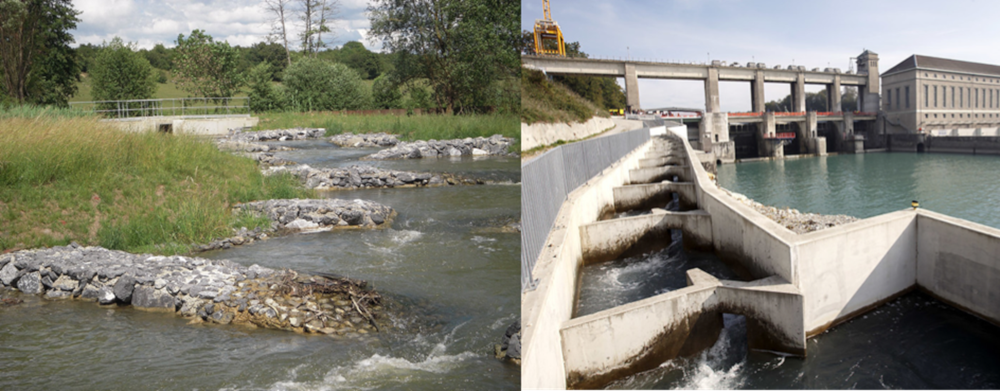
Fig. 5: Fish passes. Chatel Chehery bypass river(photo Benoit Caby), successive basin pass at Chancy-Pougny (photo Keystone)
Any obstacle to migration leads to a considerable decrease in amphibiotic populations and the confinement of holobiotic species to small portions of the watercourse. The blockage of fish passage is resolved by the installation of fish passage devices: fish passes (Figure 5), fish locks or fish lifts (Figure 6), capture and transfer by creels. These devices can be equipped with visualization and counting devices to control their efficiency.
In addition to migration blockage, there is also mortality in the turbines during the downstream migration. This mortality is, globally, all the more important when dealing with high head turbines. Pelton turbines, however, where the mortality is 100%, do not pose a problem because they are, in principle, installed on rivers that do not support migratory species. Mortality in Francis and Kaplan turbines is highly variable depending on the species and on parameters such as the size of the turbines, their speed of rotation, the layout of the hydraulic conduit and its components such as pre-distributor, distributor and turbine runner. In the case of cascade developments, this mortality is cumulative at each site, leading to high rates (Figure 7).This mortality is a long-standing concern, but more recently numerous studies have led to a better understanding of the phenomena involved and to improvements in turbine design by manufacturers to reduce mortality. Although these developments are leading to an increase in costs, they are often consistent with an improvement in performance: increased efficiency and reduced cavitation.
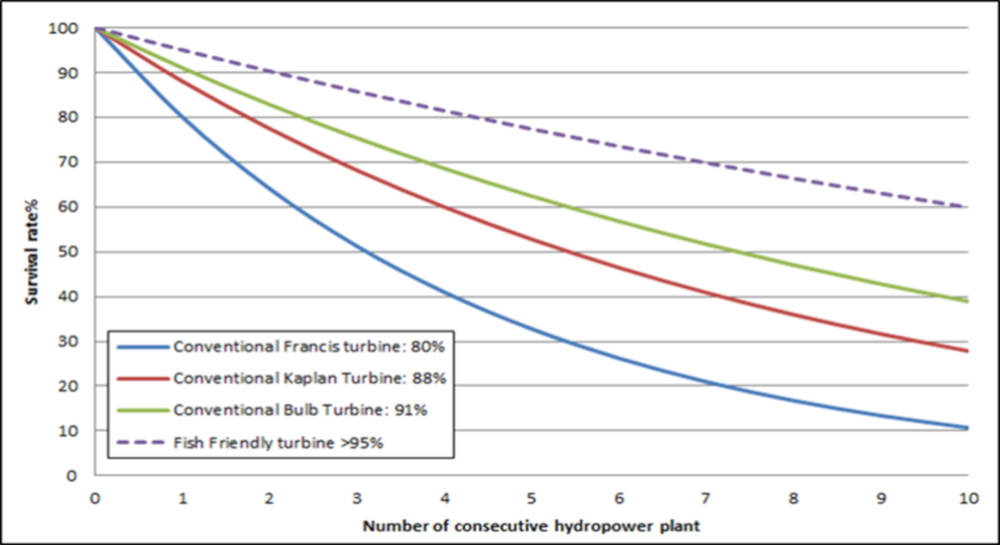
Fig. 7: Survival rate as a function of turbine type and number of developments. [Source: https://michelsabourin.scenari-community.org/SimTurb/co/1siteWeb.html]
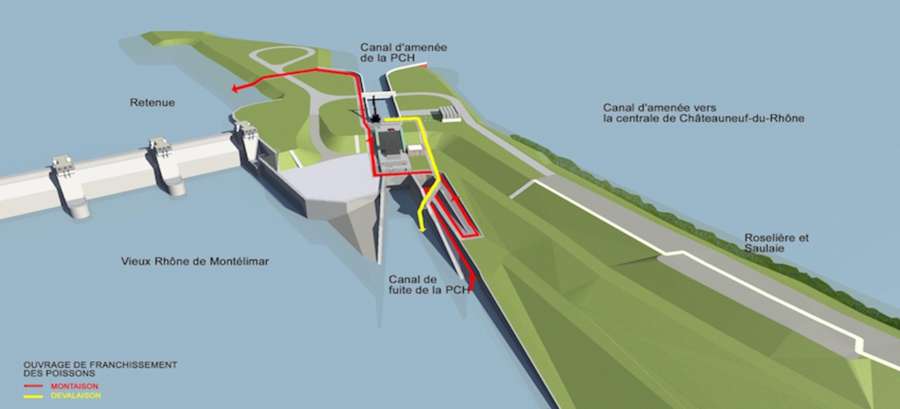
Fig. 8: Restoration of fish continuity: small hydroelectric power plant with upstream and downstream structures. [Source: Photo © CNR]
6. Consequences on flora
The impoundment of the reservoir can lead to the disappearance of protected or endemic species, as the submergence of the vegetation leads to its decomposition and the release of methane [12]. Conversely, in some arid regions, the creation of the reservoir can lead to the creation of oases useful to wildlife.
In some cases, new aquatic vegetation may develop, such as water hyacinth in tropical regions or reeds in temperate regions. Different types of algae can also proliferate in an uncontrolled manner. This proliferation of aquatic vegetation has negative consequences such as habitat degradation for most aquatic species, proliferation of insects that spread diseases, hindrance to navigation or fishing or obstruction of water intake screens. The development of this type of vegetation represents a strong constraint for the operation: removal by mechanical means, containment by drome.
7. Societal impacts
Directly, or indirectly through their environmental impacts, hydroelectric developments also affect human activities in a negative or positive way.
7.1. Population displacement
They represent the most violently felt consequence. The limitation of flooded areas makes it possible to limit the displacement of the population and the relocation must be carried out in consultation with the populations concerned. Projects must avoid affecting the territory of indigenous populations whose displacement would mean the definitive loss of a civilisation, a way of life and a unique cultural wealth (Read: The development of Sélingué in Mali).
7.2. Modifications to ways of life
Numerous human activities linked to the watercourse often represent an essential means of subsistence for the riparian populations: fishing, irrigation, navigation. The construction of the development can thus lead to a loss of income for these populations as well as to a significant modification of their way of life, which it is important to evaluate in order to fully compensate them.
The creation of large reservoirs can also remove communication routes such as roads or railways, which, if not replaced, will bring additional constraints to the local populations. Conversely, the construction of the dam may create a new means of crossing the river.
In many cases, the construction of large dams requires the mobilisation of many workers, several thousand for the largest structures, who are not available locally. The presence, even temporary, of these populations, many of whom are single, sometimes permanently disrupts the lives of local populations (Read: The impact of hydroelectricity in the Beaufortain).
The creation of a large reservoir can generate local climatic phenomena, in particular fog or rain.
Areas of stagnant water, in tropical regions, can also stimulate, locally, the proliferation of insects propagating diseases such as malaria. They can also favour the emergence of diseases such as dysentery or cholera. Preventive measures and information for the population must be taken to avoid this type of consequence.
7.3. Other effects
Many hydropower schemes are in fact so-called “multi-purpose” schemes, either because they were designed as such from the outset, or because their presence has allowed for uses other than electricity production. Their uses are therefore not limited to the production of electricity. They are also used to:
- flood control: the capacity of the reservoir allows to temporarily store the volume of water of the peak of the flood to release it progressively once the maximum flow has passed;
- low-water support, which ensures a minimum flow in the watercourse in order to preserve the aquatic environments and the quality of water, possibly for other uses such as navigation, leisure or agricultural uses;
- for navigation, dams to regulate the flow of the watercourse and to maintain water lines which ensure a sufficient draught throughout the year, as well as speeds compatible with navigation;
- a water reserve for agricultural, industrial or drinking water supply purposes.
More specifically, many reservoirs allow the development of tourism and water sports activities, bringing a new and sometimes welcome economic activity to certain regions (Figure 9).
- all these activities may be affected, positively or negatively, by other consequences of development, in the form of loss of heritage value of natural, archaeological, historical or religious sites;
- a significant modification of the landscape, especially in the case of low-head hydroelectric schemes, associated with the opening up of rivers to navigation, whereas the works (adduction galleries, penstocks, hydroelectric power stations) of high-head schemes are often entirely underground and totally invisible from the outside.
Conclusion
Hydropower still has a significant development potential in the world, particularly in Asia and Africa. Because of its renewable nature, its low GHG emissions and its technical advantages in terms of flexibility and energy storage, it represents an essential means of decarbonising electricity production and supporting the development of intermittent renewable energies, which are essential in the fight against climate change. That’s why it is essential to develop our knowledge of the environmental consequences of projects so that we can carry out environmentally friendly developments.
The choice of site for a hydroelectric facility is the first step in achieving this environmental friendliness and compatibility with GHG reduction objectives. A judicious choice of site associated with measures to eliminate, reduce and compensate for the effects will make it possible to achieve an environmentally friendly and socially acceptable project. No compensation measure can make acceptable a project built on a site resulting from an inadequate choice.
The reduction of the flooded area is also an important factor in limiting the negative consequences, as is the choice of an appropriate operating method which must minimize the consequences downstream of the development (Figure 10).
Bibliography
[1]: Peteuil Christophe (2018). Sustainable management of sediment fluxes in the Rhône river cascade. Hydrolink 4/2018.
[2]: Ramos F. et al. (2009). Methane stocks in tropical hydropower reservoirs as a potential energy source.
[3]: Chanudet V. et al. (2011). Gross CO2 and CH4 emissions from the Nam Ngum and Nam Leuk sub-tropical reservoirs in Lao PDR. Science of the Total Environment, 409 (24), 5382-5391. ISSN 0048-9697
[4]: Mendonça R., Barros N., Vidal L., Pacheco F., Kosten S. and Roland F. (2012). Greenhouse Gas Emissions from Hydroelectric Reservoirs: What Knowledge Do We Have and What is Lacking? in Greenhouse Gases – Emission, Measurement and Management, Dr Guoxiang Liu (Ed.) p. 55-78. ISBN: 978- 953-51-0323-3.
[5]: Level Y., Richard P. (2011). Small hydroelectric power plant of Rochemaure: valorisation of instream flow and fish passage. La Houille Blanche n°6, p. 17-21. Available here:http://wikhydro.developpement-durable.gouv.fr/index.php/Petite_centrale_hydroélectrique_de_Rochemaure_:_valorisation_du_débit_réservé_et_franchissement_piscicole
References
[2] The European Eco-Drilling project aims to reduce GHG emissions from this type of work by 50%.
[3] Borges A. et al. (2015). Globally significant greenhouse-gas emissions from African inland waters.
[4] Prior deforestation is not a priori a good solution. Beyond the economic criterion, the means to be implemented are themselves high GHG emitters and the duration of such an operation would not avoid the regrowth of many plants before submersion.
[5] CIRAIG study cited above.
[6] Kemenes A., Forsberg B., Melack J. (2007). Methane release below a tropical hydroelectric dam. Geophysical research letters, vol.34.
[7] Chanudet V., Descloux S., Harby A., Sundt H., Hansen B. H., Brakstad O., Serca D., GuérinFrédéric. (2011). Gross CO2 and CH4 emissions from the Nam Ngum and Nam Leuk sub-tropical reservoirs in Lao PDR. Science of the Total Environment, 409 (24), 5382-5391. ISSN 0048-9697
[8] Source: Intergovernmental Panel on Climate Change (IPCC)
[9] Ramos F. et al. (2009). Methane stocks in tropical hydropower reservoirs as a potential energy source.
[10] http://www.planseisme.fr/-Dossier-Quand-l-Homme-fait-trembler-la-Terre-.html
[11] In France, the 1919 hydroelectricity law already introduced this notion of instream flow. In 2020, Law No2006-1772 of 30 December 2006 (known as the LEMA: Law on Water and Aquatic Environments) defines the rules for setting this flow.
[12] Point dealt with above in 2.
[13] https://www.hydropower.org/gres, https://www.hydropower.org/topics/featured/hydropower-sustainability-assessment-protocol
The Encyclopedia of Energy is published by the Association of Environment and Energy Encyclopedias (A3E)(www.a3e.fr), contractually linked to Grenoble Alpes University and Grenoble INP, and sponsored by the Academy of Sciences.
To cite this article, please mention the author’s name, the title of the article and its URL on the Encyclopedia of Energy website.
The articles in the Encyclopedia of Energy are licensed under the terms of the Creative Commons Attribution-Noncommercial-No Derivative Works 4.0 International License.